Mechanism of transcriptional bursting in bacteria
- PMID: 25036631
- PMCID: PMC4105854
- DOI: 10.1016/j.cell.2014.05.038
Mechanism of transcriptional bursting in bacteria
Abstract
Transcription of highly expressed genes has been shown to occur in stochastic bursts. But the origin of such ubiquitous phenomenon has not been understood. Here, we present the mechanism in bacteria. We developed a high-throughput, in vitro, single-molecule assay to follow transcription on individual DNA templates in real time. We showed that positive supercoiling buildup on a DNA segment by transcription slows down transcription elongation and eventually stops transcription initiation. Transcription can be resumed upon gyrase binding to the DNA segment. Furthermore, using single-cell mRNA counting fluorescence in situ hybridization (FISH), we found that duty cycles of transcriptional bursting depend on the intracellular gyrase concentration. Together, these findings prove that transcriptional bursting of highly expressed genes in bacteria is primarily caused by reversible gyrase dissociation from and rebinding to a DNA segment, changing the supercoiling level of the segment.
Copyright © 2014 Elsevier Inc. All rights reserved.
Figures
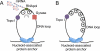
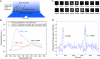
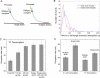
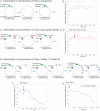
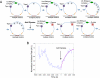
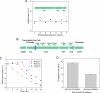
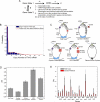
Comment in
-
A new twist on transcriptional bursting.Cell. 2014 Jul 17;158(2):241-242. doi: 10.1016/j.cell.2014.06.042. Cell. 2014. PMID: 25036624 Free PMC article.
-
Transcription: Unwinding transcriptional bursting.Nat Rev Genet. 2014 Sep;15(9):574. doi: 10.1038/nrg3800. Epub 2014 Aug 5. Nat Rev Genet. 2014. PMID: 25091870 No abstract available.
Similar articles
-
Rates of gyrase supercoiling and transcription elongation control supercoil density in a bacterial chromosome.PLoS Genet. 2012;8(8):e1002845. doi: 10.1371/journal.pgen.1002845. Epub 2012 Aug 16. PLoS Genet. 2012. PMID: 22916023 Free PMC article.
-
Dissecting the in vivo dynamics of transcription locking due to positive supercoiling buildup.Biochim Biophys Acta Gene Regul Mech. 2020 May;1863(5):194515. doi: 10.1016/j.bbagrm.2020.194515. Epub 2020 Feb 27. Biochim Biophys Acta Gene Regul Mech. 2020. PMID: 32113983
-
Inhibition of the gyrA promoter by transcription-coupled DNA supercoiling in Escherichia coli.Sci Rep. 2018 Oct 3;8(1):14759. doi: 10.1038/s41598-018-33089-4. Sci Rep. 2018. PMID: 30282997 Free PMC article.
-
DNA supercoiling and transcription in bacteria: a two-way street.BMC Mol Cell Biol. 2019 Jul 18;20(1):26. doi: 10.1186/s12860-019-0211-6. BMC Mol Cell Biol. 2019. PMID: 31319794 Free PMC article. Review.
-
The mechanism of negative DNA supercoiling: a cascade of DNA-induced conformational changes prepares gyrase for strand passage.DNA Repair (Amst). 2014 Apr;16:23-34. doi: 10.1016/j.dnarep.2014.01.011. Epub 2014 Feb 22. DNA Repair (Amst). 2014. PMID: 24674625 Review.
Cited by
-
Comparison of bias and resolvability in single-cell and single-transcript methods.Commun Biol. 2021 Jun 2;4(1):659. doi: 10.1038/s42003-021-02138-6. Commun Biol. 2021. PMID: 34079048 Free PMC article.
-
Mapping catalytically engaged TOP2B in neurons reveals the principles of topoisomerase action within the genome.Cell Rep. 2024 Feb 27;43(2):113809. doi: 10.1016/j.celrep.2024.113809. Epub 2024 Feb 19. Cell Rep. 2024. PMID: 38377005 Free PMC article.
-
Transcriptional refractoriness is dependent on core promoter architecture.Nat Commun. 2015 Apr 8;6:6753. doi: 10.1038/ncomms7753. Nat Commun. 2015. PMID: 25851692
-
First Responders Shape a Prompt and Sharp NF-κB-Mediated Transcriptional Response to TNF-α.iScience. 2020 Sep 4;23(9):101529. doi: 10.1016/j.isci.2020.101529. eCollection 2020 Sep 25. iScience. 2020. PMID: 33083759 Free PMC article.
-
Precision design of stable genetic circuits carried in highly-insulated E. coli genomic landing pads.Mol Syst Biol. 2020 Aug;16(8):e9584. doi: 10.15252/msb.20209584. Mol Syst Biol. 2020. PMID: 32812710 Free PMC article.
References
-
- Bai L, Santangelo TJ, Wang MD. Single-molecule analysis of RNA polymerase transcription. Annu Rev Biophys Biomol Struct. 2006;35:343–360. - PubMed
-
- Baker TA, Funnell BE, Kornberg A. Helicase action of dnaB protein during replication from the Escherichia coli chromosomal origin in vitro. J Biol Chem. 1987;262:6877–6885. - PubMed
-
- Billingsley DJ, Bonass WA, Crampton N, Kirkham J, Thomson NH. Single-molecule studies of DNA transcription using atomic force microscopy. Phys Biol. 2012;9:021001. - PubMed
-
- Blake WJ, M KA, Cantor CR, Collins JJ. Noise in eukaryotic gene expression. Nature. 2003;422:633–637. - PubMed
Publication types
MeSH terms
Substances
Grants and funding
LinkOut - more resources
Full Text Sources
Other Literature Sources