Enhancing astrocytic lysosome biogenesis facilitates Aβ clearance and attenuates amyloid plaque pathogenesis
- PMID: 25031402
- PMCID: PMC4099542
- DOI: 10.1523/JNEUROSCI.3788-13.2014
Enhancing astrocytic lysosome biogenesis facilitates Aβ clearance and attenuates amyloid plaque pathogenesis
Abstract
In sporadic Alzheimer's disease (AD), impaired Aβ removal contributes to elevated extracellular Aβ levels that drive amyloid plaque pathogenesis. Extracellular proteolysis, export across the blood-brain barrier, and cellular uptake facilitate physiologic Aβ clearance. Astrocytes can take up and degrade Aβ, but it remains unclear whether this function is insufficient in AD or can be enhanced to accelerate Aβ removal. Additionally, age-related dysfunction of lysosomes, the major degradative organelles wherein Aβ localizes after uptake, has been implicated in amyloid plaque pathogenesis. We tested the hypothesis that enhancing lysosomal function in astrocytes with transcription factor EB (TFEB), a master regulator of lysosome biogenesis, would promote Aβ uptake and catabolism and attenuate plaque pathogenesis. Exogenous TFEB localized to the nucleus with transcriptional induction of lysosomal biogenesis and function in vitro. This resulted in significantly accelerated uptake of exogenously applied Aβ42, with increased localization to and degradation within lysosomes in C17.2 cells and primary astrocytes, indicating that TFEB is sufficient to coordinately enhance uptake, trafficking, and degradation of Aβ. Stereotactic injection of adeno-associated viral particles carrying TFEB driven by a glial fibrillary acidic protein promoter was used to achieve astrocyte-specific expression in the hippocampus of APP/PS1 transgenic mice. Exogenous TFEB localized to astrocyte nuclei and enhanced lysosome function, resulting in reduced Aβ levels and shortened half-life in the brain interstitial fluid and reduced amyloid plaque load in the hippocampus compared with control virus-injected mice. Therefore, activation of TFEB in astrocytes is an effective strategy to restore adequate Aβ removal and counter amyloid plaque pathogenesis in AD.
Keywords: Alzheimer's disease; TFEB; amyloid; astrocytes; lysosomes.
Copyright © 2014 the authors 0270-6474/14/349607-14$15.00/0.
Figures
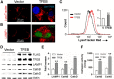
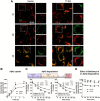
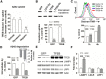
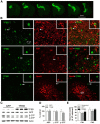
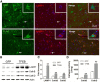
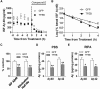
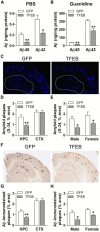
Similar articles
-
Neuronal-Targeted TFEB Accelerates Lysosomal Degradation of APP, Reducing Aβ Generation and Amyloid Plaque Pathogenesis.J Neurosci. 2015 Sep 2;35(35):12137-51. doi: 10.1523/JNEUROSCI.0705-15.2015. J Neurosci. 2015. PMID: 26338325 Free PMC article.
-
Deacetylation of TFEB promotes fibrillar Aβ degradation by upregulating lysosomal biogenesis in microglia.Protein Cell. 2016 Jun;7(6):417-33. doi: 10.1007/s13238-016-0269-2. Epub 2016 May 21. Protein Cell. 2016. PMID: 27209302 Free PMC article.
-
Gypenoside XVII Enhances Lysosome Biogenesis and Autophagy Flux and Accelerates Autophagic Clearance of Amyloid-β through TFEB Activation.J Alzheimers Dis. 2016 Apr 5;52(3):1135-50. doi: 10.3233/JAD-160096. J Alzheimers Dis. 2016. PMID: 27060963
-
TFEB in Alzheimer's disease: From molecular mechanisms to therapeutic implications.Neurobiol Dis. 2022 Oct 15;173:105855. doi: 10.1016/j.nbd.2022.105855. Epub 2022 Aug 27. Neurobiol Dis. 2022. PMID: 36031168 Review.
-
The dual and emerging role of physical exercise-induced TFEB activation in the protection against Alzheimer's disease.J Cell Physiol. 2023 May;238(5):954-965. doi: 10.1002/jcp.31005. Epub 2023 Apr 3. J Cell Physiol. 2023. PMID: 37013375 Review.
Cited by
-
SIRT1 facilitates amyloid beta peptide degradation by upregulating lysosome number in primary astrocytes.Neural Regen Res. 2018 Nov;13(11):2005-2013. doi: 10.4103/1673-5374.239449. Neural Regen Res. 2018. PMID: 30233076 Free PMC article.
-
Oleoylethanolamide facilitates PPARα and TFEB signaling and attenuates Aβ pathology in a mouse model of Alzheimer's disease.Mol Neurodegener. 2023 Aug 15;18(1):56. doi: 10.1186/s13024-023-00648-x. Mol Neurodegener. 2023. PMID: 37580742 Free PMC article.
-
The role of MiT/TFE family members in autophagy regulation.Curr Top Biochem Res. 2021;22:151-159. Curr Top Biochem Res. 2021. PMID: 35663368 Free PMC article.
-
Graptopetalum paraguayense Extract Ameliorates Proteotoxicity in Aging and Age-Related Diseases in Model Systems.Nutrients. 2021 Nov 29;13(12):4317. doi: 10.3390/nu13124317. Nutrients. 2021. PMID: 34959868 Free PMC article.
-
Associations of TFEB Gene Polymorphisms With Cognitive Function in Rural Chinese Population.Front Aging Neurosci. 2021 Dec 14;13:757992. doi: 10.3389/fnagi.2021.757992. eCollection 2021. Front Aging Neurosci. 2021. PMID: 34970136 Free PMC article.
References
-
- Avrahami L, Farfara D, Shaham-Kol M, Vassar R, Frenkel D, Eldar-Finkelman H. Inhibition of glycogen synthase kinase-3 ameliorates beta-amyloid pathology and restores lysosomal acidification and mammalian target of rapamycin activity in the Alzheimer disease mouse model: in vivo and in vitro studies. J Biol Chem. 2013;288:1295–1306. doi: 10.1074/jbc.M112.409250. - DOI - PMC - PubMed
Publication types
MeSH terms
Substances
Grants and funding
LinkOut - more resources
Full Text Sources
Other Literature Sources