Rassf5 and Ndr kinases regulate neuronal polarity through Par3 phosphorylation in a novel pathway
- PMID: 24928906
- PMCID: PMC4132390
- DOI: 10.1242/jcs.146696
Rassf5 and Ndr kinases regulate neuronal polarity through Par3 phosphorylation in a novel pathway
Abstract
The morphology and polarized growth of cells depend on pathways that control the asymmetric distribution of regulatory factors. The evolutionarily conserved Ndr kinases play important roles in cell polarity and morphogenesis in yeast and invertebrates but it is unclear whether they perform a similar function in mammalian cells. Here, we analyze the function of mammalian Ndr1 and Ndr2 (also known as STK38 or STK38L, respectively) in the establishment of polarity in neurons. We show that they act downstream of the tumor suppressor Rassf5 and upstream of the polarity protein Par3 (also known as PARD3). Rassf5 and Ndr1 or Ndr2 are required during the polarization of hippocampal neurons to prevent the formation of supernumerary axons. Mechanistically, the Ndr kinases act by phosphorylating Par3 at Ser383 to inhibit its interaction with dynein, thereby polarizing the distribution of Par3 and reinforcing axon specification. Our results identify a novel Rassf5-Ndr-Par3 signaling cascade that regulates the transport of Par3 during the establishment of neuronal polarity. Their role in neuronal polarity suggests that Ndr kinases perform a conserved function as regulators of cell polarity.
Keywords: Axon formation; Cell polarity; Ndr kinases; Par.
© 2014. Published by The Company of Biologists Ltd.
Figures
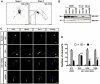
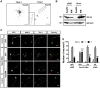
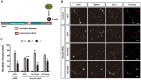
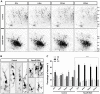
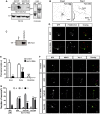
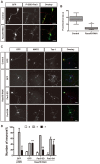
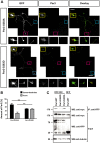
Similar articles
-
Phosphorylation of the par polarity complex protein Par3 at serine 962 is mediated by aurora a and regulates its function in neuronal polarity.J Biol Chem. 2009 Nov 27;284(48):33571-9. doi: 10.1074/jbc.M109.055897. Epub 2009 Oct 6. J Biol Chem. 2009. PMID: 19812038 Free PMC article.
-
Par3 is essential for the establishment of planar cell polarity of inner ear hair cells.Proc Natl Acad Sci U S A. 2019 Mar 12;116(11):4999-5008. doi: 10.1073/pnas.1816333116. Epub 2019 Feb 27. Proc Natl Acad Sci U S A. 2019. PMID: 30814219 Free PMC article.
-
PAR3-PAR6-atypical PKC polarity complex proteins in neuronal polarization.Cell Mol Life Sci. 2018 Aug;75(15):2735-2761. doi: 10.1007/s00018-018-2828-6. Epub 2018 Apr 25. Cell Mol Life Sci. 2018. PMID: 29696344 Free PMC article. Review.
-
The signaling adaptor GAB1 regulates cell polarity by acting as a PAR protein scaffold.Mol Cell. 2012 Aug 10;47(3):469-83. doi: 10.1016/j.molcel.2012.06.037. Mol Cell. 2012. PMID: 22883624 Free PMC article.
-
The NDR/LATS protein kinases in immunology and cancer biology.Semin Cancer Biol. 2018 Feb;48:104-114. doi: 10.1016/j.semcancer.2017.04.010. Epub 2017 Jun 1. Semin Cancer Biol. 2018. PMID: 28579171 Review.
Cited by
-
Transcriptomic analysis and novel insights into lens fibre cell differentiation regulated by Gata3.Open Biol. 2019 Dec;9(12):190220. doi: 10.1098/rsob.190220. Epub 2019 Dec 18. Open Biol. 2019. PMID: 31847788 Free PMC article.
-
PTEN-GSK3β-MOB1 axis controls neurite outgrowth in vitro and in vivo.Cell Mol Life Sci. 2018 Dec;75(23):4445-4464. doi: 10.1007/s00018-018-2890-0. Epub 2018 Aug 1. Cell Mol Life Sci. 2018. PMID: 30069702 Free PMC article.
-
The loss of the kinases SadA and SadB results in early neuronal apoptosis and a reduced number of progenitors.PLoS One. 2018 Apr 26;13(4):e0196698. doi: 10.1371/journal.pone.0196698. eCollection 2018. PLoS One. 2018. PMID: 29698519 Free PMC article.
-
The guanine nucleotide exchange factor Arhgef7/βPix promotes axon formation upstream of TC10.Sci Rep. 2018 Jun 11;8(1):8811. doi: 10.1038/s41598-018-27081-1. Sci Rep. 2018. PMID: 29891904 Free PMC article.
-
Regulation of cytoskeletal dynamics by redox signaling and oxidative stress: implications for neuronal development and trafficking.Front Cell Neurosci. 2015 Sep 30;9:381. doi: 10.3389/fncel.2015.00381. eCollection 2015. Front Cell Neurosci. 2015. PMID: 26483635 Free PMC article. Review.
References
Publication types
MeSH terms
Substances
Grants and funding
LinkOut - more resources
Full Text Sources
Other Literature Sources
Molecular Biology Databases
Research Materials