In Planta Recognition of a Double-Stranded RNA Synthesis Protein Complex by a Potexviral RNA Silencing Suppressor
- PMID: 24879427
- PMCID: PMC4079376
- DOI: 10.1105/tpc.113.120535
In Planta Recognition of a Double-Stranded RNA Synthesis Protein Complex by a Potexviral RNA Silencing Suppressor
Abstract
RNA silencing plays an important antiviral role in plants and invertebrates. To counteract antiviral RNA silencing, most plant viruses have evolved viral suppressors of RNA silencing (VSRs). TRIPLE GENE BLOCK PROTEIN1 (TGBp1) of potexviruses is a well-characterized VSR, but the detailed mechanism by which it suppresses RNA silencing remains unclear. We demonstrate that transgenic expression of TGBp1 of plantago asiatica mosaic virus (PlAMV) induced developmental abnormalities in Arabidopsis thaliana similar to those observed in mutants of SUPPRESSOR OF GENE SILENCING3 (SGS3) and RNA-DEPENDENT RNA POLYMERASE6 (RDR6) required for the trans-acting small interfering RNA synthesis pathway. PlAMV-TGBp1 inhibits SGS3/RDR6-dependent double-stranded RNA synthesis in the trans-acting small interfering RNA pathway. TGBp1 interacts with SGS3 and RDR6 and coaggregates with SGS3/RDR6 bodies, which are normally dispersed in the cytoplasm. In addition, TGBp1 forms homooligomers, whose formation coincides with TGBp1 aggregation with SGS3/RDR6 bodies. These results reveal the detailed molecular function of TGBp1 as a VSR and shed new light on the SGS3/RDR6-dependent double-stranded RNA synthesis pathway as another general target of VSRs.
© 2014 American Society of Plant Biologists. All rights reserved.
Figures
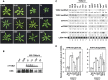
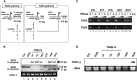
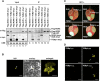
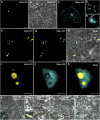
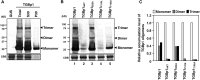
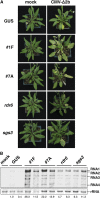
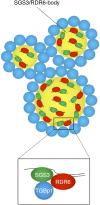
Similar articles
-
The Potyvirus Silencing Suppressor Protein VPg Mediates Degradation of SGS3 via Ubiquitination and Autophagy Pathways.J Virol. 2016 Dec 16;91(1):e01478-16. doi: 10.1128/JVI.01478-16. Print 2017 Jan 1. J Virol. 2016. PMID: 27795417 Free PMC article.
-
Turnip crinkle virus-encoded suppressor of RNA silencing interacts with Arabidopsis SGS3 to enhance virus infection.Mol Plant Pathol. 2023 Feb;24(2):154-166. doi: 10.1111/mpp.13282. Epub 2022 Nov 26. Mol Plant Pathol. 2023. PMID: 36433724 Free PMC article.
-
A small peptide inhibits siRNA amplification in plants by mediating autophagic degradation of SGS3/RDR6 bodies.EMBO J. 2021 Aug 2;40(15):e108050. doi: 10.15252/embj.2021108050. Epub 2021 Jun 22. EMBO J. 2021. PMID: 34155657 Free PMC article.
-
Plantago asiatica mosaic virus: An emerging plant virus causing necrosis in lilies and a new model RNA virus for molecular research.Mol Plant Pathol. 2022 Oct;23(10):1401-1414. doi: 10.1111/mpp.13243. Epub 2022 Jul 20. Mol Plant Pathol. 2022. PMID: 35856603 Free PMC article. Review.
-
Virus counterdefense: diverse strategies for evading the RNA-silencing immunity.Annu Rev Microbiol. 2006;60:503-31. doi: 10.1146/annurev.micro.60.080805.142205. Annu Rev Microbiol. 2006. PMID: 16768647 Free PMC article. Review.
Cited by
-
Viral RNase3 Co-Localizes and Interacts with the Antiviral Defense Protein SGS3 in Plant Cells.PLoS One. 2016 Jul 8;11(7):e0159080. doi: 10.1371/journal.pone.0159080. eCollection 2016. PLoS One. 2016. PMID: 27391019 Free PMC article.
-
The phytoplasmal virulence factor TENGU causes plant sterility by downregulating of the jasmonic acid and auxin pathways.Sci Rep. 2014 Dec 10;4:7399. doi: 10.1038/srep07399. Sci Rep. 2014. PMID: 25492247 Free PMC article.
-
Dynamin-related protein 2 interacts with the membrane-associated methyltransferase domain of plantago asiatica mosaic virus replicase and promotes viral replication.Virus Res. 2023 Jul 2;331:199128. doi: 10.1016/j.virusres.2023.199128. Epub 2023 May 14. Virus Res. 2023. PMID: 37149224 Free PMC article.
-
Interaction between Brassica yellows virus silencing suppressor P0 and plant SKP1 facilitates stability of P0 in vivo against degradation by proteasome and autophagy pathways.New Phytol. 2019 May;222(3):1458-1473. doi: 10.1111/nph.15702. Epub 2019 Feb 26. New Phytol. 2019. PMID: 30664234 Free PMC article.
-
TYMV and TRV infect Arabidopsis thaliana by expressing weak suppressors of RNA silencing and inducing host RNASE THREE LIKE1.PLoS Pathog. 2023 Jan 25;19(1):e1010482. doi: 10.1371/journal.ppat.1010482. eCollection 2023 Jan. PLoS Pathog. 2023. PMID: 36696453 Free PMC article.
References
-
- Adenot X., Elmayan T., Lauressergues D., Boutet S., Bouché N., Gasciolli V., Vaucheret H. (2006). DRB4-dependent TAS3 trans-acting siRNAs control leaf morphology through AGO7. Curr. Biol. 16: 927–932 - PubMed
-
- Allen E., Howell M.D. (2010). miRNAs in the biogenesis of trans-acting siRNAs in higher plants. Semin. Cell Dev. Biol. 21: 798–804 - PubMed
-
- Allen E., Xie Z., Gustafson A.M., Carrington J.C. (2005). MicroRNA-directed phasing during trans-acting siRNA biogenesis in plants. Cell 121: 207–221 - PubMed
LinkOut - more resources
Full Text Sources
Other Literature Sources
Molecular Biology Databases
Research Materials