Achieving high-frequency optical control of synaptic transmission
- PMID: 24872574
- PMCID: PMC4035530
- DOI: 10.1523/JNEUROSCI.4694-13.2014
Achieving high-frequency optical control of synaptic transmission
Abstract
The optogenetic tool channelrhodopsin-2 (ChR2) is widely used to excite neurons to study neural circuits. Previous optogenetic studies of synapses suggest that light-evoked synaptic responses often exhibit artificial synaptic depression, which has been attributed to either the inability of ChR2 to reliably fire presynaptic axons or to ChR2 elevating the probability of release by depolarizing presynaptic boutons. Here, we compare light-evoked and electrically evoked synaptic responses for high-frequency stimulation at three synapses in the mouse brain. At synapses from Purkinje cells to deep cerebellar nuclei neurons (PC→DCN), light- and electrically evoked synaptic currents were remarkably similar for ChR2 expressed transgenically or with adeno-associated virus (AAV) expression vectors. For hippocampal CA3→CA1 synapses, AAV expression vectors of serotype 1, 5, and 8 led to light-evoked synaptic currents that depressed much more than electrically evoked currents, even though ChR2 could fire axons reliably at up to 50 Hz. The disparity between optical and electrical stimulation was eliminated when ChR2 was expressed transgenically or with AAV9. For cerebellar granule cell to stellate cell (grc→SC) synapses, AAV1 also led to artificial synaptic depression and AAV9 provided superior performance. Artificial synaptic depression also occurred when stimulating over presynaptic boutons, rather than axons, at CA3→CA1 synapses, but not at PC→DCN synapses. These findings indicate that ChR2 expression methods and light stimulation techniques influence synaptic responses in a neuron-specific manner. They also identify pitfalls associated with using ChR2 to study synapses and suggest an approach that allows optogenetics to be applied in a manner that helps to avoid potential complications.
Keywords: AAV; channelrhodopsin; optogenetics; short-term plasticity; synapse.
Copyright © 2014 the authors 0270-6474/14/347704-11$15.00/0.
Figures
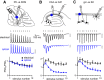
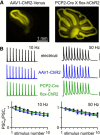
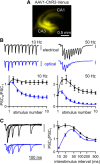
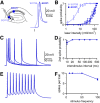
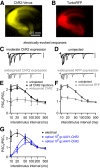
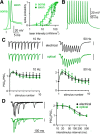
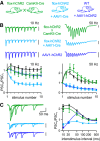
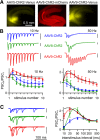
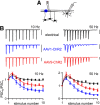
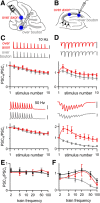
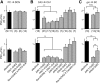
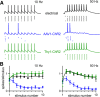
Similar articles
-
A Slow Short-Term Depression at Purkinje to Deep Cerebellar Nuclear Neuron Synapses Supports Gain-Control and Linear Encoding over Second-Long Time Windows.J Neurosci. 2020 Jul 29;40(31):5937-5953. doi: 10.1523/JNEUROSCI.2078-19.2020. Epub 2020 Jun 17. J Neurosci. 2020. PMID: 32554551 Free PMC article.
-
Optogenetic approaches to characterize the long-range synaptic pathways from the hypothalamus to brain stem autonomic nuclei.J Neurosci Methods. 2012 Sep 30;210(2):238-46. doi: 10.1016/j.jneumeth.2012.07.022. Epub 2012 Aug 7. J Neurosci Methods. 2012. PMID: 22890236 Free PMC article.
-
Long-Term Depression Induced by Optogenetically Driven Nociceptive Inputs to Trigeminal Nucleus Caudalis or Headache Triggers.J Neurosci. 2018 Aug 22;38(34):7529-7540. doi: 10.1523/JNEUROSCI.3032-17.2018. Epub 2018 Jul 27. J Neurosci. 2018. PMID: 30054391 Free PMC article.
-
Channelrhodopsin as a tool to investigate synaptic transmission and plasticity.Exp Physiol. 2011 Jan;96(1):34-9. doi: 10.1113/expphysiol.2009.051219. Epub 2010 Jun 18. Exp Physiol. 2011. PMID: 20562296 Review.
-
Optogenetics: Illuminating the Future of Hearing Restoration and Understanding Auditory Perception.Curr Gene Ther. 2024;24(3):208-216. doi: 10.2174/0115665232269742231213110937. Curr Gene Ther. 2024. PMID: 38676313 Review.
Cited by
-
Organization of Cortical and Thalamic Input to Inhibitory Neurons in Mouse Motor Cortex.J Neurosci. 2022 Oct 26;42(43):8095-8112. doi: 10.1523/JNEUROSCI.0950-22.2022. Epub 2022 Sep 14. J Neurosci. 2022. PMID: 36104281 Free PMC article.
-
Efferent synaptic transmission at the vestibular type II hair cell synapse.J Neurophysiol. 2020 Aug 1;124(2):360-374. doi: 10.1152/jn.00143.2020. Epub 2020 Jul 1. J Neurophysiol. 2020. PMID: 32609559 Free PMC article.
-
Altered Chloride Homeostasis Decreases the Action Potential Threshold and Increases Hyperexcitability in Hippocampal Neurons.eNeuro. 2018 Jan 23;4(6):ENEURO.0172-17.2017. doi: 10.1523/ENEURO.0172-17.2017. eCollection 2017 Nov-Dec. eNeuro. 2018. PMID: 29379872 Free PMC article.
-
Synaptic function and plasticity in identified inhibitory inputs onto VTA dopamine neurons.Eur J Neurosci. 2018 May;47(10):1208-1218. doi: 10.1111/ejn.13879. Epub 2018 Mar 13. Eur J Neurosci. 2018. PMID: 29480954 Free PMC article.
-
Excitation and Inhibition Delays within a Feedforward Inhibitory Pathway Modulate Cerebellar Purkinje Cell Output in Mice.J Neurosci. 2023 Aug 16;43(33):5905-5917. doi: 10.1523/JNEUROSCI.0091-23.2023. Epub 2023 Jul 26. J Neurosci. 2023. PMID: 37495382 Free PMC article.
References
Publication types
MeSH terms
Substances
Grants and funding
LinkOut - more resources
Full Text Sources
Other Literature Sources