Moving towards a paradigm: common mechanisms of chemotactic signaling in Dictyostelium and mammalian leukocytes
- PMID: 24846395
- PMCID: PMC4162842
- DOI: 10.1007/s00018-014-1638-8
Moving towards a paradigm: common mechanisms of chemotactic signaling in Dictyostelium and mammalian leukocytes
Abstract
Chemotaxis, or directed migration of cells along a chemical gradient, is a highly coordinated process that involves gradient sensing, motility, and polarity. Most of our understanding of chemotaxis comes from studies of cells undergoing amoeboid-type migration, in particular the social amoeba Dictyostelium discoideum and leukocytes. In these amoeboid cells the molecular events leading to directed migration can be conceptually divided into four interacting networks: receptor/G protein, signal transduction, cytoskeleton, and polarity. The signal transduction network occupies a central position in this scheme as it receives direct input from the receptor/G protein network, as well as feedback from the cytoskeletal and polarity networks. Multiple overlapping modules within the signal transduction network transmit the signals to the actin cytoskeleton network leading to biased pseudopod protrusion in the direction of the gradient. The overall architecture of the networks, as well as the individual signaling modules, is remarkably conserved between Dictyostelium and mammalian leukocytes, and the similarities and differences between the two systems are the subject of this review.
Figures
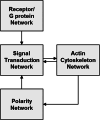
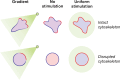
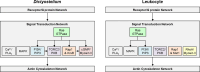
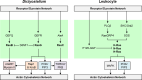
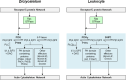
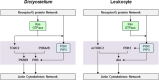
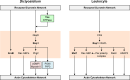
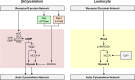
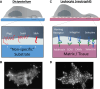
Similar articles
-
Phosphorylation of chemoattractant receptors regulates chemotaxis, actin reorganization and signal relay.J Cell Sci. 2013 Oct 15;126(Pt 20):4614-26. doi: 10.1242/jcs.122952. Epub 2013 Jul 31. J Cell Sci. 2013. PMID: 23902692 Free PMC article.
-
Imaging GPCR-Mediated Signal Events Leading to Chemotaxis and Phagocytosis.Methods Mol Biol. 2021;2304:207-220. doi: 10.1007/978-1-0716-1402-0_11. Methods Mol Biol. 2021. PMID: 34028719
-
ELMO proteins transduce G protein-coupled receptor signal to control reorganization of actin cytoskeleton in chemotaxis of eukaryotic cells.Small GTPases. 2019 Jul;10(4):271-279. doi: 10.1080/21541248.2017.1318816. Epub 2017 Aug 14. Small GTPases. 2019. PMID: 28641070 Free PMC article. Review.
-
Phosphoinositides in chemotaxis.Subcell Biochem. 2012;59:217-54. doi: 10.1007/978-94-007-3015-1_7. Subcell Biochem. 2012. PMID: 22374092 Review.
-
Mechanisms of leukocyte motility and chemotaxis.Curr Opin Immunol. 1994 Feb;6(1):113-24. doi: 10.1016/0952-7915(94)90042-6. Curr Opin Immunol. 1994. PMID: 8172673 Review.
Cited by
-
Spatial gradient sensing and chemotaxis via excitability in Dictyostelium discoideum.Phys Rev E. 2020 Jun;101(6-1):062410. doi: 10.1103/PhysRevE.101.062410. Phys Rev E. 2020. PMID: 32688583 Free PMC article.
-
Heterotrimeric G-protein shuttling via Gip1 extends the dynamic range of eukaryotic chemotaxis.Proc Natl Acad Sci U S A. 2016 Apr 19;113(16):4356-61. doi: 10.1073/pnas.1516767113. Epub 2016 Apr 4. Proc Natl Acad Sci U S A. 2016. PMID: 27044073 Free PMC article.
-
Copine A Interacts with Actin Filaments and Plays a Role in Chemotaxis and Adhesion.Cells. 2019 Jul 21;8(7):758. doi: 10.3390/cells8070758. Cells. 2019. PMID: 31330887 Free PMC article.
-
Actin Waves and Dynamic Patterning of the Plasma Membrane.Yale J Biol Med. 2019 Sep 20;92(3):397-411. eCollection 2019 Sep. Yale J Biol Med. 2019. PMID: 31543704 Free PMC article.
-
Coordination of Receptor Tyrosine Kinase Signaling and Interfacial Tension Dynamics Drives Radial Intercalation and Tube Elongation.Dev Cell. 2018 Apr 9;45(1):67-82.e6. doi: 10.1016/j.devcel.2018.03.011. Dev Cell. 2018. PMID: 29634937 Free PMC article.
References
-
- Richardson BE, Lehmann R (2010) Mechanisms guiding primordial germ cell migration: strategies from different organisms. Nat Rev Mol Cell Biol 11 (1):37-49. doi:http://www.nature.com/nrm/journal/v11/n1/suppinfo/nrm2815_S1.html - PMC - PubMed
-
- Kolaczkowska E, Kubes P. Neutrophil recruitment and function in health and inflammation. Nat Rev Immunol. 2013;13(3):159–175. - PubMed
Publication types
MeSH terms
Substances
Grants and funding
LinkOut - more resources
Full Text Sources
Other Literature Sources