Distinct stages of the translation elongation cycle revealed by sequencing ribosome-protected mRNA fragments
- PMID: 24842990
- PMCID: PMC4052883
- DOI: 10.7554/eLife.01257
Distinct stages of the translation elongation cycle revealed by sequencing ribosome-protected mRNA fragments
Abstract
During translation elongation, the ribosome ratchets along its mRNA template, incorporating each new amino acid and translocating from one codon to the next. The elongation cycle requires dramatic structural rearrangements of the ribosome. We show here that deep sequencing of ribosome-protected mRNA fragments reveals not only the position of each ribosome but also, unexpectedly, its particular stage of the elongation cycle. Sequencing reveals two distinct populations of ribosome footprints, 28-30 nucleotides and 20-22 nucleotides long, representing translating ribosomes in distinct states, differentially stabilized by specific elongation inhibitors. We find that the balance of small and large footprints varies by codon and is correlated with translation speed. The ability to visualize conformational changes in the ribosome during elongation, at single-codon resolution, provides a new way to study the detailed kinetics of translation and a new probe with which to identify the factors that affect each step in the elongation cycle.DOI: http://dx.doi.org/10.7554/eLife.01257.001.
Keywords: ribosome; ribosome profiling; translation.
Copyright © 2014, Lareau et al.
Conflict of interest statement
The authors declare that no competing interests exist.
Figures
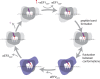
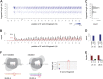
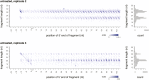
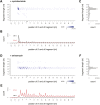
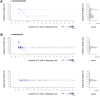
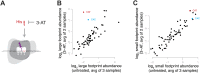
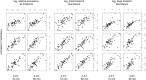
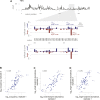
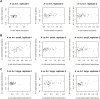

Similar articles
-
Insights into the mechanisms of eukaryotic translation gained with ribosome profiling.Nucleic Acids Res. 2017 Jan 25;45(2):513-526. doi: 10.1093/nar/gkw1190. Epub 2016 Dec 6. Nucleic Acids Res. 2017. PMID: 27923997 Free PMC article. Review.
-
High-Resolution Ribosome Profiling for Determining Ribosome Functional States During Translation Elongation.Methods Mol Biol. 2022;2428:173-186. doi: 10.1007/978-1-0716-1975-9_11. Methods Mol Biol. 2022. PMID: 35171480
-
High-Resolution Ribosome Profiling Defines Discrete Ribosome Elongation States and Translational Regulation during Cellular Stress.Mol Cell. 2019 Mar 7;73(5):959-970.e5. doi: 10.1016/j.molcel.2018.12.009. Epub 2019 Jan 24. Mol Cell. 2019. PMID: 30686592 Free PMC article.
-
Translation complex profile sequencing to study the in vivo dynamics of mRNA-ribosome interactions during translation initiation, elongation and termination.Nat Protoc. 2017 Apr;12(4):697-731. doi: 10.1038/nprot.2016.189. Epub 2017 Mar 2. Nat Protoc. 2017. PMID: 28253237
-
How Messenger RNA and Nascent Chain Sequences Regulate Translation Elongation.Annu Rev Biochem. 2018 Jun 20;87:421-449. doi: 10.1146/annurev-biochem-060815-014818. Annu Rev Biochem. 2018. PMID: 29925264 Free PMC article. Review.
Cited by
-
Sex Differences in Primary HIV Infection: Revisiting the Role of TLR7-Driven Type 1 IFN Production by Plasmacytoid Dendritic Cells in Women.Front Immunol. 2021 Aug 27;12:729233. doi: 10.3389/fimmu.2021.729233. eCollection 2021. Front Immunol. 2021. PMID: 34512664 Free PMC article. Review.
-
Next generation sequencing technologies to address aberrant mRNA translation in cancer.NAR Cancer. 2024 May 15;6(2):zcae024. doi: 10.1093/narcan/zcae024. eCollection 2024 Jun. NAR Cancer. 2024. PMID: 38751936 Free PMC article. Review.
-
Insights into the mechanisms of eukaryotic translation gained with ribosome profiling.Nucleic Acids Res. 2017 Jan 25;45(2):513-526. doi: 10.1093/nar/gkw1190. Epub 2016 Dec 6. Nucleic Acids Res. 2017. PMID: 27923997 Free PMC article. Review.
-
Regulated Ire1-dependent mRNA decay requires no-go mRNA degradation to maintain endoplasmic reticulum homeostasis in S. pombe.Elife. 2017 Sep 25;6:e29216. doi: 10.7554/eLife.29216. Elife. 2017. PMID: 28945192 Free PMC article.
-
eIF5A facilitates translation termination globally and promotes the elongation of many non polyproline-specific tripeptide sequences.Nucleic Acids Res. 2017 Jul 7;45(12):7326-7338. doi: 10.1093/nar/gkx479. Nucleic Acids Res. 2017. PMID: 28549188 Free PMC article.
References
Publication types
MeSH terms
Substances
Grants and funding
LinkOut - more resources
Full Text Sources
Other Literature Sources
Molecular Biology Databases