GroEL/ES chaperonin modulates the mechanism and accelerates the rate of TIM-barrel domain folding
- PMID: 24813614
- PMCID: PMC4071350
- DOI: 10.1016/j.cell.2014.03.038
GroEL/ES chaperonin modulates the mechanism and accelerates the rate of TIM-barrel domain folding
Abstract
The GroEL/ES chaperonin system functions as a protein folding cage. Many obligate substrates of GroEL share the (βα)8 TIM-barrel fold, but how the chaperonin promotes folding of these proteins is not known. Here, we analyzed the folding of DapA at peptide resolution using hydrogen/deuterium exchange and mass spectrometry. During spontaneous folding, all elements of the DapA TIM barrel acquire structure simultaneously in a process associated with a long search time. In contrast, GroEL/ES accelerates folding more than 30-fold by catalyzing segmental structure formation in the TIM barrel. Segmental structure formation is also observed during the fast spontaneous folding of a structural homolog of DapA from a bacterium that lacks GroEL/ES. Thus, chaperonin independence correlates with folding properties otherwise enforced by protein confinement in the GroEL/ES cage. We suggest that folding catalysis by GroEL/ES is required by a set of proteins to reach native state at a biologically relevant timescale, avoiding aggregation or degradation.
Copyright © 2014 Elsevier Inc. All rights reserved.
Figures
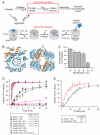
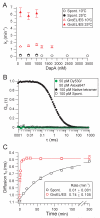
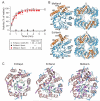
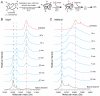
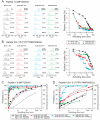
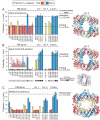
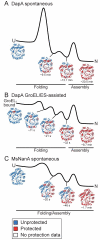
Similar articles
-
Proteome-wide analysis of chaperonin-dependent protein folding in Escherichia coli.Cell. 2005 Jul 29;122(2):209-20. doi: 10.1016/j.cell.2005.05.028. Cell. 2005. PMID: 16051146
-
Efficient Catalysis of Protein Folding by GroEL/ES of the Obligate Chaperonin Substrate MetF.J Mol Biol. 2020 Mar 27;432(7):2304-2318. doi: 10.1016/j.jmb.2020.02.031. Epub 2020 Mar 2. J Mol Biol. 2020. PMID: 32135190
-
Active cage mechanism of chaperonin-assisted protein folding demonstrated at single-molecule level.J Mol Biol. 2014 Jul 29;426(15):2739-54. doi: 10.1016/j.jmb.2014.04.018. Epub 2014 May 6. J Mol Biol. 2014. PMID: 24816391
-
GroEL-assisted protein folding: does it occur within the chaperonin inner cavity?Int J Mol Sci. 2009 May 12;10(5):2066-2083. doi: 10.3390/ijms10052066. Int J Mol Sci. 2009. PMID: 19564940 Free PMC article. Review.
-
Molecular chaperone GroEL/ES: unfolding and refolding processes.Biochemistry (Mosc). 2013 Dec;78(13):1405-14. doi: 10.1134/S0006297913130038. Biochemistry (Mosc). 2013. PMID: 24490731 Review.
Cited by
-
From chaperonins to Rubisco assembly and metabolic repair.Protein Sci. 2017 Dec;26(12):2324-2333. doi: 10.1002/pro.3309. Epub 2017 Oct 10. Protein Sci. 2017. PMID: 28960553 Free PMC article. Review.
-
The case for defined protein folding pathways.Proc Natl Acad Sci U S A. 2017 Aug 1;114(31):8253-8258. doi: 10.1073/pnas.1706196114. Epub 2017 Jun 19. Proc Natl Acad Sci U S A. 2017. PMID: 28630329 Free PMC article.
-
The diverse and expanding role of mass spectrometry in structural and molecular biology.EMBO J. 2016 Dec 15;35(24):2634-2657. doi: 10.15252/embj.201694818. Epub 2016 Oct 26. EMBO J. 2016. PMID: 27797822 Free PMC article. Review.
-
Comparative genomic analysis of mollicutes with and without a chaperonin system.PLoS One. 2018 Feb 13;13(2):e0192619. doi: 10.1371/journal.pone.0192619. eCollection 2018. PLoS One. 2018. PMID: 29438383 Free PMC article.
-
Synthesis and folding of a mirror-image enzyme reveals ambidextrous chaperone activity.Proc Natl Acad Sci U S A. 2014 Aug 12;111(32):11679-84. doi: 10.1073/pnas.1410900111. Epub 2014 Jul 28. Proc Natl Acad Sci U S A. 2014. PMID: 25071217 Free PMC article.
References
-
- Azia A, Unger R, Horovitz A. What distinguishes GroEL substrates from other Escherichia coli proteins? FEBS J. 2012;279:543–550. - PubMed
-
- Baumketner A, Jewett A, Shea JE. Effects of confinement in chaperonin assisted protein folding: Rate enhancement by decreasing the roughness of the folding energy landscape. J. Mol. Biol. 2003;332:701–713. - PubMed
-
- Brinker A, Pfeifer G, Kerner MJ, Naylor DJ, Hartl FU, Hayer-Hartl M. Dual function of protein confinement in chaperonin-assisted protein folding. Cell. 2001;107:223–233. - PubMed
Publication types
MeSH terms
Substances
Grants and funding
LinkOut - more resources
Full Text Sources
Other Literature Sources
Molecular Biology Databases
Research Materials