Single-vesicle architecture of synaptobrevin2 in astrocytes
- PMID: 24807050
- PMCID: PMC4344122
- DOI: 10.1038/ncomms4780
Single-vesicle architecture of synaptobrevin2 in astrocytes
Abstract
Exocytic transmitter release is regulated by the SNARE complex, which contains a vesicular protein, synaptobrevin2 (Sb2). However, Sb2 vesicular arrangement is unclear. Here we use super-resolution fluorescence microscopy to study the prevalence and distribution of endogenous and exogenous Sb2 in single vesicles of astrocytes, the most abundant glial cells in the brain. We tag Sb2 protein at C- and N termini with a pair of fluorophores, which allows us to determine the Sb2 length and geometry. To estimate total number of Sb2 proteins per vesicle and the quantity necessary for the formation of fusion pores, we treat cells with ATP to stimulate Ca2+-dependent exocytosis, increase intracellular alkalinity to enhance the fluorescence presentation of yellow-shifted pHluorin (YpH), appended to the vesicle lumen domain of Sb2, and perform photobleaching of YpH fluorophores. Fluorescence intensity analysis reveals that the total number of endogenous Sb2 units or molecules per vesicle is ≤25.
Conflict of interest statement
Figures
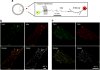
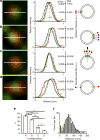
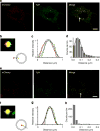
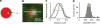
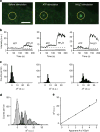
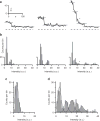
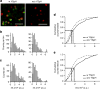
Similar articles
-
Loose excitation-secretion coupling in astrocytes.Glia. 2016 May;64(5):655-67. doi: 10.1002/glia.22920. Epub 2015 Sep 11. Glia. 2016. PMID: 26358496 Free PMC article. Review.
-
Astrocytic vesicles and gliotransmitters: Slowness of vesicular release and synaptobrevin2-laden vesicle nanoarchitecture.Neuroscience. 2016 May 26;323:67-75. doi: 10.1016/j.neuroscience.2015.02.033. Epub 2015 Feb 26. Neuroscience. 2016. PMID: 25727638 Review.
-
Synaptobrevin2-expressing vesicles in rat astrocytes: insights into molecular characterization, dynamics and exocytosis.J Physiol. 2006 Feb 1;570(Pt 3):567-82. doi: 10.1113/jphysiol.2005.094052. Epub 2005 Dec 1. J Physiol. 2006. PMID: 16322057 Free PMC article.
-
Ketamine Inhibits ATP-Evoked Exocytotic Release of Brain-Derived Neurotrophic Factor from Vesicles in Cultured Rat Astrocytes.Mol Neurobiol. 2016 Dec;53(10):6882-6896. doi: 10.1007/s12035-015-9562-y. Epub 2015 Dec 12. Mol Neurobiol. 2016. PMID: 26660497
-
Probing single molecule mechanical interactions of syntaxin 1A with native synaptobrevin 2 residing on a secretory vesicle.Cell Calcium. 2022 Jun;104:102570. doi: 10.1016/j.ceca.2022.102570. Epub 2022 Mar 11. Cell Calcium. 2022. PMID: 35314381 Free PMC article.
Cited by
-
Imaging Synaptic Density: The Next Holy Grail of Neuroscience?Front Neurosci. 2022 Mar 25;16:796129. doi: 10.3389/fnins.2022.796129. eCollection 2022. Front Neurosci. 2022. PMID: 35401097 Free PMC article. Review.
-
Chemically Functionalized Water-Soluble Single-Walled Carbon Nanotubes Obstruct Vesicular/Plasmalemmal Recycling in Astrocytes Down-Stream of Calcium Ions.Cells. 2020 Jul 1;9(7):1597. doi: 10.3390/cells9071597. Cells. 2020. PMID: 32630262 Free PMC article.
-
Substance use and spine density: a systematic review and meta-analysis of preclinical studies.Mol Psychiatry. 2024 Sep;29(9):2873-2885. doi: 10.1038/s41380-024-02519-3. Epub 2024 Apr 2. Mol Psychiatry. 2024. PMID: 38561468
-
Loose excitation-secretion coupling in astrocytes.Glia. 2016 May;64(5):655-67. doi: 10.1002/glia.22920. Epub 2015 Sep 11. Glia. 2016. PMID: 26358496 Free PMC article. Review.
-
A novel method for culturing stellate astrocytes reveals spatially distinct Ca2+ signaling and vesicle recycling in astrocytic processes.J Gen Physiol. 2017 Jan;149(1):149-170. doi: 10.1085/jgp.201611607. Epub 2016 Dec 1. J Gen Physiol. 2017. PMID: 27908976 Free PMC article.
References
-
- Nedergaard M, Ransom B, Goldman SA. New roles for astrocytes: redefining the functional architecture of the brain. Trends Neurosci. 2003;26:523–530. - PubMed
-
- Halassa MM, Fellin T, Haydon PG. The tripartite synapse: roles for gliotransmission in health and disease. Trends Mol Med. 2007;13:54–63. - PubMed
-
- Wienisch M, Klingauf J. Vesicular proteins exocytosed and subsequently retrieved by compensatory endocytosis are nonidentical. Nat Neurosci. 2006;9:1019–1027. - PubMed
-
- Hua Y, et al. A readily retrievable pool of synaptic vesicles. Nat Neurosci. 2011;14:833–839. - PubMed
Publication types
MeSH terms
Substances
Grants and funding
LinkOut - more resources
Full Text Sources
Other Literature Sources
Miscellaneous