Proteolytic cleavage of Opa1 stimulates mitochondrial inner membrane fusion and couples fusion to oxidative phosphorylation
- PMID: 24703695
- PMCID: PMC4018240
- DOI: 10.1016/j.cmet.2014.03.011
Proteolytic cleavage of Opa1 stimulates mitochondrial inner membrane fusion and couples fusion to oxidative phosphorylation
Erratum in
- Cell Metab. 2014 May 6;19(5):891
Abstract
Mitochondrial fusion is essential for maintenance of mitochondrial function. The mitofusin GTPases control mitochondrial outer membrane fusion, whereas the dynamin-related GTPase Opa1 mediates inner membrane fusion. We show that mitochondrial inner membrane fusion is tuned by the level of oxidative phosphorylation (OXPHOS), whereas outer membrane fusion is insensitive. Consequently, cells from patients with pathogenic mtDNA mutations show a selective defect in mitochondrial inner membrane fusion. In elucidating the molecular mechanism of OXPHOS-stimulated fusion, we uncover that real-time proteolytic processing of Opa1 stimulates mitochondrial inner membrane fusion. OXPHOS-stimulated mitochondrial fusion operates through Yme1L, which cleaves Opa1 more efficiently under high OXPHOS conditions. Engineered cleavage of Opa1 is sufficient to mediate inner membrane fusion, regardless of respiratory state. Proteolytic cleavage therefore stimulates the membrane fusion activity of Opa1, and this feature is exploited to dynamically couple mitochondrial fusion to cellular metabolism.
Copyright © 2014 Elsevier Inc. All rights reserved.
Figures
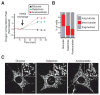
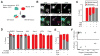
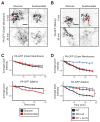
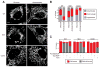
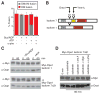
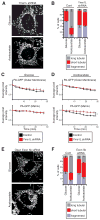
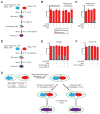
Similar articles
-
The short variant of the mitochondrial dynamin OPA1 maintains mitochondrial energetics and cristae structure.J Biol Chem. 2017 Apr 28;292(17):7115-7130. doi: 10.1074/jbc.M116.762567. Epub 2017 Mar 15. J Biol Chem. 2017. PMID: 28298442 Free PMC article.
-
Loss of Drp1 function alters OPA1 processing and changes mitochondrial membrane organization.Exp Cell Res. 2009 Aug 1;315(13):2165-80. doi: 10.1016/j.yexcr.2009.04.016. Epub 2009 May 3. Exp Cell Res. 2009. PMID: 19409380
-
OPA1 regulates respiratory supercomplexes assembly: The role of mitochondrial swelling.Mitochondrion. 2020 Mar;51:30-39. doi: 10.1016/j.mito.2019.11.006. Epub 2019 Dec 20. Mitochondrion. 2020. PMID: 31870826 Free PMC article.
-
Molecular mechanism of mitochondrial membrane fusion.Biochim Biophys Acta. 2006 May-Jun;1763(5-6):482-9. doi: 10.1016/j.bbamcr.2006.02.003. Epub 2006 Mar 9. Biochim Biophys Acta. 2006. PMID: 16571363 Review.
-
OPA1 processing in cell death and disease - the long and short of it.J Cell Sci. 2016 Jun 15;129(12):2297-306. doi: 10.1242/jcs.159186. Epub 2016 May 17. J Cell Sci. 2016. PMID: 27189080 Review.
Cited by
-
Cellular pyrimidine imbalance triggers mitochondrial DNA-dependent innate immunity.Nat Metab. 2021 May;3(5):636-650. doi: 10.1038/s42255-021-00385-9. Epub 2021 Apr 26. Nat Metab. 2021. PMID: 33903774 Free PMC article.
-
Phenotypic plasticity of vascular smooth muscle cells in vascular calcification: Role of mitochondria.Front Cardiovasc Med. 2022 Oct 12;9:972836. doi: 10.3389/fcvm.2022.972836. eCollection 2022. Front Cardiovasc Med. 2022. PMID: 36312244 Free PMC article. Review.
-
Regulation of Mitoflash Biogenesis and Signaling by Mitochondrial Dynamics.Sci Rep. 2016 Sep 13;6:32933. doi: 10.1038/srep32933. Sci Rep. 2016. PMID: 27623243 Free PMC article.
-
In situ architecture of Opa1-dependent mitochondrial cristae remodeling.EMBO J. 2024 Feb;43(3):391-413. doi: 10.1038/s44318-024-00027-2. Epub 2024 Jan 15. EMBO J. 2024. PMID: 38225406 Free PMC article.
-
New roles for mitochondrial proteases in health, ageing and disease.Nat Rev Mol Cell Biol. 2015 Jun;16(6):345-59. doi: 10.1038/nrm3984. Epub 2015 May 13. Nat Rev Mol Cell Biol. 2015. PMID: 25970558 Review.
References
-
- Baracca A, Solaini G, Sgarbi G, Lenaz G, Baruzzi A, Schapira AH, Martinuzzi A, Carelli V. Severe impairment of complex I-driven adenosine triphosphate synthesis in leber hereditary optic neuropathy cybrids. Archives of neurology. 2005;62:730–736. - PubMed
-
- Chan DC. Fusion and fission: interlinked processes critical for mitochondrial health. Annual review of genetics. 2012;46:265–287. - PubMed
-
- Chance B, Williams GR. Respiratory enzymes in oxidative phosphorylation. III. The steady state. J Biol Chem. 1955;217:409–427. - PubMed
Publication types
MeSH terms
Substances
Grants and funding
LinkOut - more resources
Full Text Sources
Other Literature Sources
Molecular Biology Databases
Research Materials