Review: biogenesis of the multifunctional lipid droplet: lipids, proteins, and sites
- PMID: 24590170
- PMCID: PMC3941045
- DOI: 10.1083/jcb.201311051
Review: biogenesis of the multifunctional lipid droplet: lipids, proteins, and sites
Abstract
Lipid droplets (LDs) are ubiquitous dynamic organelles that store and supply lipids in all eukaryotic and some prokaryotic cells for energy metabolism, membrane synthesis, and production of essential lipid-derived molecules. Interest in the organelle's cell biology has exponentially increased over the last decade due to the link between LDs and prevalent human diseases and the discovery of new and unexpected functions of LDs. As a result, there has been significant recent progress toward understanding where and how LDs are formed, and the specific lipid pathways that coordinate LD biogenesis.
Figures
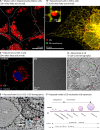
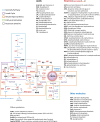
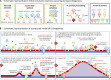
Similar articles
-
Spatial compartmentalization of lipid droplet biogenesis.Biochim Biophys Acta Mol Cell Biol Lipids. 2020 Jan;1865(1):158499. doi: 10.1016/j.bbalip.2019.07.008. Epub 2019 Jul 25. Biochim Biophys Acta Mol Cell Biol Lipids. 2020. PMID: 31352131 Free PMC article. Review.
-
Lipid droplet quantification based on iterative image processing.J Lipid Res. 2019 Jul;60(7):1333-1344. doi: 10.1194/jlr.D092841. Epub 2019 Mar 29. J Lipid Res. 2019. PMID: 30926625 Free PMC article.
-
Acyl-CoA synthetase 3 promotes lipid droplet biogenesis in ER microdomains.J Cell Biol. 2013 Dec 23;203(6):985-1001. doi: 10.1083/jcb.201305142. J Cell Biol. 2013. PMID: 24368806 Free PMC article.
-
Lipid droplets and peroxisomes: key players in cellular lipid homeostasis or a matter of fat--store 'em up or burn 'em down.Genetics. 2013 Jan;193(1):1-50. doi: 10.1534/genetics.112.143362. Genetics. 2013. PMID: 23275493 Free PMC article. Review.
-
Lipid droplet growth: regulation of a dynamic organelle.Curr Opin Cell Biol. 2017 Aug;47:9-15. doi: 10.1016/j.ceb.2017.02.002. Epub 2017 Feb 21. Curr Opin Cell Biol. 2017. PMID: 28231490 Review.
Cited by
-
Expanding roles for lipid droplets.Curr Biol. 2015 Jun 1;25(11):R470-81. doi: 10.1016/j.cub.2015.04.004. Curr Biol. 2015. PMID: 26035793 Free PMC article. Review.
-
Spatial control of lipid droplet proteins by the ERAD ubiquitin ligase Doa10.EMBO J. 2016 Aug 1;35(15):1644-55. doi: 10.15252/embj.201593106. Epub 2016 Jun 29. EMBO J. 2016. PMID: 27357570 Free PMC article.
-
A transcriptomic examination of encased rotifer embryos reveals the developmental trajectory leading to long-term dormancy; are they "animal seeds"?BMC Genomics. 2024 Jan 27;25(1):119. doi: 10.1186/s12864-024-09961-1. BMC Genomics. 2024. PMID: 38281016 Free PMC article.
-
As the fat flies: The dynamic lipid droplets of Drosophila embryos.Biochim Biophys Acta. 2015 Sep;1851(9):1156-85. doi: 10.1016/j.bbalip.2015.04.002. Epub 2015 Apr 13. Biochim Biophys Acta. 2015. PMID: 25882628 Free PMC article. Review.
-
Septin 9 induces lipid droplets growth by a phosphatidylinositol-5-phosphate and microtubule-dependent mechanism hijacked by HCV.Nat Commun. 2016 Jul 15;7:12203. doi: 10.1038/ncomms12203. Nat Commun. 2016. PMID: 27417143 Free PMC article.
References
-
- Ayciriex S., Le Guédard M., Camougrand N., Velours G., Schoene M., Leone S., Wattelet-Boyer V., Dupuy J.W., Shevchenko A., Schmitter J.M., et al. 2012. YPR139c/LOA1 encodes a novel lysophosphatidic acid acyltransferase associated with lipid droplets and involved in TAG homeostasis. Mol. Biol. Cell. 23:233–246 10.1091/mbc.E11-07-0650 - DOI - PMC - PubMed
Publication types
MeSH terms
Substances
Grants and funding
LinkOut - more resources
Full Text Sources
Other Literature Sources
Research Materials