The eukaryotic RNA exosome
- PMID: 24525139
- PMCID: PMC3985421
- DOI: 10.1016/j.sbi.2014.01.011
The eukaryotic RNA exosome
Abstract
The eukaryotic RNA exosome is an essential multi-subunit ribonuclease complex that contributes to the degradation or processing of nearly every class of RNA in both the nucleus and cytoplasm. Its nine-subunit core shares structural similarity to phosphorolytic exoribonucleases such as bacterial PNPase. PNPase and the RNA exosome core feature a central channel that can accommodate single stranded RNA although unlike PNPase, the RNA exosome core is devoid of ribonuclease activity. Instead, the core associates with Rrp44, an endoribonuclease and processive 3'→5' exoribonuclease, and Rrp6, a distributive 3'→5' exoribonuclease. Recent biochemical and structural studies suggest that the exosome core is essential because it coordinates Rrp44 and Rrp6 recruitment, RNA can pass through the central channel, and the association with the core modulates Rrp44 and Rrp6 activities.
Copyright © 2014 Elsevier Ltd. All rights reserved.
Figures
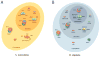
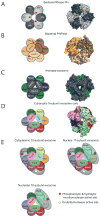
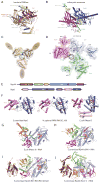
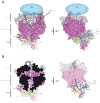
Similar articles
-
The Rrp6 C-terminal domain binds RNA and activates the nuclear RNA exosome.Nucleic Acids Res. 2017 Jan 25;45(2):846-860. doi: 10.1093/nar/gkw1152. Epub 2016 Nov 29. Nucleic Acids Res. 2017. PMID: 27899565 Free PMC article.
-
Exo- and endoribonucleolytic activities of yeast cytoplasmic and nuclear RNA exosomes are dependent on the noncatalytic core and central channel.Mol Cell. 2012 Oct 12;48(1):133-44. doi: 10.1016/j.molcel.2012.07.012. Epub 2012 Aug 16. Mol Cell. 2012. PMID: 22902556 Free PMC article.
-
Structure of an Rrp6-RNA exosome complex bound to poly(A) RNA.Nature. 2014 Jul 24;511(7510):435-9. doi: 10.1038/nature13406. Epub 2014 Jul 6. Nature. 2014. PMID: 25043052 Free PMC article.
-
Structural components and architectures of RNA exosomes.Adv Exp Med Biol. 2010;702:9-28. Adv Exp Med Biol. 2010. PMID: 21618871 Free PMC article. Review.
-
Rrp6, Rrp47 and cofactors of the nuclear exosome.Adv Exp Med Biol. 2010;702:91-104. Adv Exp Med Biol. 2010. PMID: 21618877 Review.
Cited by
-
Substrate discrimination and quality control require each catalytic activity of TRAMP and the nuclear RNA exosome.Proc Natl Acad Sci U S A. 2021 Apr 6;118(14):e2024846118. doi: 10.1073/pnas.2024846118. Proc Natl Acad Sci U S A. 2021. PMID: 33782132 Free PMC article.
-
Exosome complex components 1 and 2 are vital for early mammalian development.Gene Expr Patterns. 2024 Mar;51:119346. doi: 10.1016/j.gep.2023.119346. Epub 2023 Nov 7. Gene Expr Patterns. 2024. PMID: 37940010
-
The cryptic unstable transcripts are associated with developmentally regulated gene expression in blood-stage Plasmodium falciparum.RNA Biol. 2020 Jun;17(6):828-842. doi: 10.1080/15476286.2020.1732032. Epub 2020 Feb 27. RNA Biol. 2020. PMID: 32079470 Free PMC article.
-
Extracellular vesicles have variable dose-dependent effects on cultured draining cells in the eye.J Cell Mol Med. 2018 Mar;22(3):1992-2000. doi: 10.1111/jcmm.13505. Epub 2018 Feb 7. J Cell Mol Med. 2018. PMID: 29411534 Free PMC article.
-
Post-transcriptional regulation shapes the transcriptome of quiescent budding yeast.Nucleic Acids Res. 2024 Feb 9;52(3):1043-1063. doi: 10.1093/nar/gkad1147. Nucleic Acids Res. 2024. PMID: 38048329 Free PMC article.
References
-
- Porrua O, Libri D. RNA quality control in the nucleus: the Angels’ share of RNA. Biochim Biophys Acta. 2013;1829:604–611. - PubMed
-
- Chlebowski A, Lubas M, Jensen TH, Dziembowski A. RNA decay machines: the exosome. Biochim Biophys Acta. 2013;1829:552–560. - PubMed
-
- Symmons MF, Jones GH, Luisi BF. A duplicated fold is the structural basis for polynucleotide phosphorylase catalytic activity, processivity, and regulation. Structure. 2000;8:1215–1226. - PubMed
-
- Liu Q, Greimann JC, Lima CD. Reconstitution, activities, and structure of the eukaryotic RNA exosome. Cell. 2006;127:1223–1237. - PubMed
Publication types
MeSH terms
Substances
Grants and funding
LinkOut - more resources
Full Text Sources
Other Literature Sources