Alu elements shape the primate transcriptome by cis-regulation of RNA editing
- PMID: 24485196
- PMCID: PMC4053975
- DOI: 10.1186/gb-2014-15-2-r28
Alu elements shape the primate transcriptome by cis-regulation of RNA editing
Abstract
Background: RNA editing by adenosine to inosine deamination is a widespread phenomenon, particularly frequent in the human transcriptome, largely due to the presence of inverted Alu repeats and their ability to form double-stranded structures--a requisite for ADAR editing. While several hundred thousand editing sites have been identified within these primate-specific repeats, the function of Alu-editing has yet to be elucidated.
Results: We show that inverted Alu repeats, expressed in the primate brain, can induce site-selective editing in cis on sites located several hundred nucleotides from the Alu elements. Furthermore, a computational analysis, based on available RNA-seq data, finds that site-selective editing occurs significantly closer to edited Alu elements than expected. These targets are poorly edited upon deletion of the editing inducers, as well as in homologous transcripts from organisms lacking Alus. Sequences surrounding sites near edited Alus in UTRs, have been subjected to a lesser extent of evolutionary selection than those far from edited Alus, indicating that their editing generally depends on cis-acting Alus. Interestingly, we find an enrichment of primate-specific editing within encoded sequence or the UTRs of zinc finger-containing transcription factors.
Conclusions: We propose a model whereby primate-specific editing is induced by adjacent Alu elements that function as recruitment elements for the ADAR editing enzymes. The enrichment of site-selective editing with potentially functional consequences on the expression of transcription factors indicates that editing contributes more profoundly to the transcriptomic regulation and repertoire in primates than previously thought.
Figures
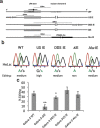
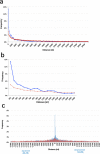
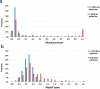
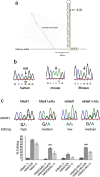
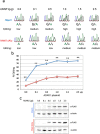
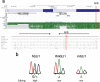
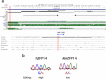
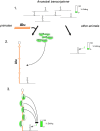
Similar articles
-
RNA editing of non-coding RNA and its role in gene regulation.Biochimie. 2015 Oct;117:22-7. doi: 10.1016/j.biochi.2015.05.020. Epub 2015 Jun 5. Biochimie. 2015. PMID: 26051678 Review.
-
A-to-I RNA editing occurs at over a hundred million genomic sites, located in a majority of human genes.Genome Res. 2014 Mar;24(3):365-76. doi: 10.1101/gr.164749.113. Epub 2013 Dec 17. Genome Res. 2014. PMID: 24347612 Free PMC article.
-
One hundred million adenosine-to-inosine RNA editing sites: hearing through the noise.Bioessays. 2014 Aug;36(8):730-5. doi: 10.1002/bies.201400055. Epub 2014 May 30. Bioessays. 2014. PMID: 24889193 Free PMC article.
-
ALU A-to-I RNA Editing: Millions of Sites and Many Open Questions.Methods Mol Biol. 2021;2181:149-162. doi: 10.1007/978-1-0716-0787-9_9. Methods Mol Biol. 2021. PMID: 32729079 Review.
-
Identification of widespread ultra-edited human RNAs.PLoS Genet. 2011 Oct;7(10):e1002317. doi: 10.1371/journal.pgen.1002317. Epub 2011 Oct 20. PLoS Genet. 2011. PMID: 22028664 Free PMC article.
Cited by
-
Diverse selective regimes shape genetic diversity at ADAR genes and at their coding targets.RNA Biol. 2015;12(2):149-61. doi: 10.1080/15476286.2015.1017215. RNA Biol. 2015. PMID: 25826567 Free PMC article.
-
The dynamic epitranscriptome: A to I editing modulates genetic information.Chromosoma. 2016 Mar;125(1):51-63. doi: 10.1007/s00412-015-0526-9. Epub 2015 Jul 7. Chromosoma. 2016. PMID: 26148686 Free PMC article. Review.
-
How RNA structure dictates the usage of a critical exon of spinal muscular atrophy gene.Biochim Biophys Acta Gene Regul Mech. 2019 Nov-Dec;1862(11-12):194403. doi: 10.1016/j.bbagrm.2019.07.004. Epub 2019 Jul 16. Biochim Biophys Acta Gene Regul Mech. 2019. PMID: 31323435 Free PMC article. Review.
-
A-to-I RNA editing - immune protector and transcriptome diversifier.Nat Rev Genet. 2018 Aug;19(8):473-490. doi: 10.1038/s41576-018-0006-1. Nat Rev Genet. 2018. PMID: 29692414 Review.
-
Repetitive elements regulate circular RNA biogenesis.Mob Genet Elements. 2015 May 21;5(3):1-7. doi: 10.1080/2159256X.2015.1045682. eCollection 2015 May-Jun. Mob Genet Elements. 2015. PMID: 26442181 Free PMC article.
References
Publication types
MeSH terms
Substances
LinkOut - more resources
Full Text Sources
Other Literature Sources