The metastable brain
- PMID: 24411730
- PMCID: PMC3997258
- DOI: 10.1016/j.neuron.2013.12.022
The metastable brain
Abstract
Neural ensembles oscillate across a broad range of frequencies and are transiently coupled or "bound" together when people attend to a stimulus, perceive, think, and act. This is a dynamic, self-assembling process, with parts of the brain engaging and disengaging in time. But how is it done? The theory of Coordination Dynamics proposes a mechanism called metastability, a subtle blend of integration and segregation. Tendencies for brain regions to express their individual autonomy and specialized functions (segregation, modularity) coexist with tendencies to couple and coordinate globally for multiple functions (integration). Although metastability has garnered increasing attention, it has yet to be demonstrated and treated within a fully spatiotemporal perspective. Here, we illustrate metastability in continuous neural and behavioral recordings, and we discuss theory and experiments at multiple scales, suggesting that metastable dynamics underlie the real-time coordination necessary for the brain's dynamic cognitive, behavioral, and social functions.
Copyright © 2014 Elsevier Inc. All rights reserved.
Conflict of interest statement
The authors do not have any financial conflict of interest that might be construed to influence the results or interpretation of the present manuscript.
Figures
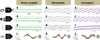
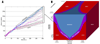
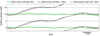
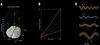
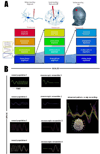
Similar articles
-
Metastable neural dynamics underlies cognitive performance across multiple behavioural paradigms.Hum Brain Mapp. 2020 Aug 15;41(12):3212-3234. doi: 10.1002/hbm.25009. Epub 2020 Apr 17. Hum Brain Mapp. 2020. PMID: 32301561 Free PMC article.
-
Brain coordination dynamics: true and false faces of phase synchrony and metastability.Prog Neurobiol. 2009 Jan 12;87(1):31-40. doi: 10.1016/j.pneurobio.2008.09.014. Epub 2008 Sep 30. Prog Neurobiol. 2009. PMID: 18938209 Free PMC article. Review.
-
Plasticity in single neuron and circuit computations.Nature. 2004 Oct 14;431(7010):789-95. doi: 10.1038/nature03011. Nature. 2004. PMID: 15483600 Review.
-
Spatiotemporal computations of an excitable and plastic brain: neuronal plasticity leads to noise-robust and noise-constructive computations.PLoS Comput Biol. 2014 Mar 20;10(3):e1003512. doi: 10.1371/journal.pcbi.1003512. eCollection 2014 Mar. PLoS Comput Biol. 2014. PMID: 24651447 Free PMC article.
-
The meaning of mammalian adult neurogenesis and the function of newly added neurons: the "small-world" network.Med Hypotheses. 2005;64(1):114-7. doi: 10.1016/j.mehy.2004.05.013. Med Hypotheses. 2005. PMID: 15533625
Cited by
-
A Broken Duet: Multistable Dynamics in Dyadic Interactions.Entropy (Basel). 2024 Aug 28;26(9):731. doi: 10.3390/e26090731. Entropy (Basel). 2024. PMID: 39330066 Free PMC article.
-
Charting moment-to-moment brain signal variability from early to late childhood.Cortex. 2016 Oct;83:51-61. doi: 10.1016/j.cortex.2016.07.006. Epub 2016 Jul 15. Cortex. 2016. PMID: 27479615 Free PMC article.
-
Rethinking segregation and integration: contributions of whole-brain modelling.Nat Rev Neurosci. 2015 Jul;16(7):430-9. doi: 10.1038/nrn3963. Epub 2015 Jun 17. Nat Rev Neurosci. 2015. PMID: 26081790 Review.
-
Adolescent Emotional Maturation through Divergent Models of Brain Organization.Front Psychol. 2016 Aug 23;7:1263. doi: 10.3389/fpsyg.2016.01263. eCollection 2016. Front Psychol. 2016. PMID: 27602012 Free PMC article.
-
Cognitive Flexibility through Metastable Neural Dynamics Is Disrupted by Damage to the Structural Connectome.J Neurosci. 2015 Jun 17;35(24):9050-63. doi: 10.1523/JNEUROSCI.4648-14.2015. J Neurosci. 2015. PMID: 26085630 Free PMC article.
References
-
- Abrams DM, Strogatz SH. Chimera states in rings of nonlocally coupled oscillators. Int J Bifurcat Chaos. 2006;16:21–37.
-
- Alba A, Marroquín JL, Arce-Santana E, Harmony T. Classification and interactive segmentation of EEG synchrony patterns. Pattern Recognit. 2010;43(2):530–544.
-
- Allen PG, Collins FS. Toward the final frontier: the human brain. Wall St J (East Ed) 2013 Apr 8; 2013.
Publication types
MeSH terms
Grants and funding
LinkOut - more resources
Full Text Sources
Other Literature Sources