Pericyte loss influences Alzheimer-like neurodegeneration in mice
- PMID: 24336108
- PMCID: PMC3945879
- DOI: 10.1038/ncomms3932
Pericyte loss influences Alzheimer-like neurodegeneration in mice
Erratum in
-
Author Correction: Pericyte loss influences Alzheimer-like neurodegeneration in mice.Nat Commun. 2023 Oct 3;14(1):6151. doi: 10.1038/s41467-023-40730-y. Nat Commun. 2023. PMID: 37788984 Free PMC article. No abstract available.
Retraction in
-
Retraction Note: Pericyte loss influences Alzheimer-like neurodegeneration in mice.Nat Commun. 2024 Apr 3;15(1):2882. doi: 10.1038/s41467-024-47285-6. Nat Commun. 2024. PMID: 38570509 Free PMC article. No abstract available.
Abstract
Pericytes are cells in the blood-brain barrier that degenerate in Alzheimer's disease (AD), a neurological disorder associated with neurovascular dysfunction, abnormal elevation of amyloid β-peptide (Aβ), tau pathology and neuronal loss. Whether pericyte degeneration can influence AD-like neurodegeneration and contribute to disease pathogenesis remains, however, unknown. Here we show that in mice overexpressing Aβ-precursor protein, pericyte loss elevates brain Aβ40 and Aβ42 levels and accelerates amyloid angiopathy and cerebral β-amyloidosis by diminishing clearance of soluble Aβ40 and Aβ42 from brain interstitial fluid prior to Aβ deposition. We further show that pericyte deficiency leads to the development of tau pathology and an early neuronal loss that is normally absent in Aβ-precursor protein transgenic mice, resulting in cognitive decline. Our data suggest that pericytes control multiple steps of AD-like neurodegeneration pathogenic cascade in Aβ-precursor protein-overexpressing mice. Therefore, pericytes may represent a novel therapeutic target to modify disease progression in AD.
Figures
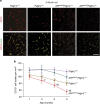
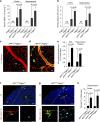
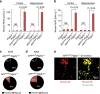
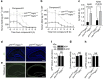
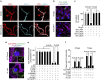
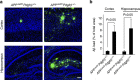
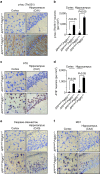
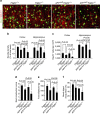
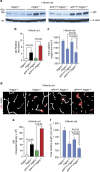
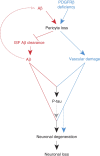
Similar articles
-
Identification of early pericyte loss and vascular amyloidosis in Alzheimer's disease retina.Acta Neuropathol. 2020 May;139(5):813-836. doi: 10.1007/s00401-020-02134-w. Epub 2020 Feb 10. Acta Neuropathol. 2020. PMID: 32043162 Free PMC article.
-
The amyloid-β degradation intermediate Aβ34 is pericyte-associated and reduced in brain capillaries of patients with Alzheimer's disease.Acta Neuropathol Commun. 2019 Dec 3;7(1):194. doi: 10.1186/s40478-019-0846-8. Acta Neuropathol Commun. 2019. PMID: 31796114 Free PMC article.
-
Pericyte implantation in the brain enhances cerebral blood flow and reduces amyloid-β pathology in amyloid model mice.Exp Neurol. 2018 Feb;300:13-21. doi: 10.1016/j.expneurol.2017.10.023. Epub 2017 Oct 26. Exp Neurol. 2018. PMID: 29106980 Free PMC article.
-
Effects of CX3CR1 and Fractalkine Chemokines in Amyloid Beta Clearance and p-Tau Accumulation in Alzheimer's Disease (AD) Rodent Models: Is Fractalkine a Systemic Biomarker for AD?Curr Alzheimer Res. 2016;13(4):403-12. doi: 10.2174/1567205013666151116125714. Curr Alzheimer Res. 2016. PMID: 26567742 Review.
-
The pericyte: a forgotten cell type with important implications for Alzheimer's disease?Brain Pathol. 2014 Jul;24(4):371-86. doi: 10.1111/bpa.12152. Brain Pathol. 2014. PMID: 24946075 Free PMC article. Review.
Cited by
-
Regulation of Endothelial and Vascular Functions by Carbon Monoxide via Crosstalk With Nitric Oxide.Front Cardiovasc Med. 2021 Apr 12;8:649630. doi: 10.3389/fcvm.2021.649630. eCollection 2021. Front Cardiovasc Med. 2021. PMID: 33912601 Free PMC article. Review.
-
Associations between Vascular Function and Tau PET Are Associated with Global Cognition and Amyloid.J Neurosci. 2020 Oct 28;40(44):8573-8586. doi: 10.1523/JNEUROSCI.1230-20.2020. Epub 2020 Oct 12. J Neurosci. 2020. PMID: 33046556 Free PMC article.
-
Transplantation of hPSC-derived pericyte-like cells promotes functional recovery in ischemic stroke mice.Nat Commun. 2020 Oct 15;11(1):5196. doi: 10.1038/s41467-020-19042-y. Nat Commun. 2020. PMID: 33060592 Free PMC article.
-
Meox2 haploinsufficiency increases neuronal cell loss in a mouse model of Alzheimer's disease.Neurobiol Aging. 2016 Jun;42:50-60. doi: 10.1016/j.neurobiolaging.2016.02.025. Epub 2016 Mar 2. Neurobiol Aging. 2016. PMID: 27143421 Free PMC article.
-
Vascular Function and Ion Channels in Alzheimer's Disease.Microcirculation. 2024 Oct;31(7):e12881. doi: 10.1111/micc.12881. Epub 2024 Aug 27. Microcirculation. 2024. PMID: 39190776 Review.
References
-
- Armulik A., Genove G. & Betsholtz C. Pericytes: developmental, physiological, and pathological perspectives, problems, and promises. Dev. Cell 21, 193–215 (2011). - PubMed
-
- Zlokovic B. V. The blood-brain barrier in health and chronic neurodegenerative disorders. Neuron 57, 178–201 (2008). - PubMed
-
- Armulik A. et al.. Pericytes regulate the blood-brain barrier. Nature 468, 557–561 (2010). - PubMed
Publication types
MeSH terms
Substances
Grants and funding
LinkOut - more resources
Full Text Sources
Other Literature Sources
Medical
Molecular Biology Databases
Miscellaneous