Synthesis, characterization, and evaluation of paclitaxel loaded in six-arm star-shaped poly(lactic-co-glycolic acid)
- PMID: 24235829
- PMCID: PMC3825676
- DOI: 10.2147/IJN.S51629
Synthesis, characterization, and evaluation of paclitaxel loaded in six-arm star-shaped poly(lactic-co-glycolic acid)
Abstract
Background: Star-shaped polymers provide more terminal groups, and are promising for application in drug-delivery systems.
Methods: A new series of six-arm star-shaped poly(lactic-co-glycolic acid) (6-s-PLGA) was synthesized by ring-opening polymerization. The structure and properties of the 6-s-PLGA were characterized by carbon-13 nuclear magnetic resonance spectroscopy, infrared spectroscopy, gel permeation chromatography, and differential scanning calorimetry. Then, paclitaxel-loaded six-arm star-shaped poly(lactic-co-glycolic acid) nanoparticles (6-s-PLGA-PTX-NPs) were prepared under the conditions optimized by the orthogonal testing. High-performance liquid chromatography was used to analyze the nanoparticles' encapsulation efficiency and drug-loading capacity, dynamic light scattering was used to determine their size and size distribution, and transmission electron microscopy was used to evaluate their morphology. The release performance of the 6-s-PLGA-PTX-NPs in vitro and the cytostatic effect of 6-s-PLGA-PTX-NPs were investigated in comparison with paclitaxel-loaded linear poly(lactic-co-glycolic acid) nanoparticles (L-PLGA-PTX-NPs).
Results: The results of carbon-13 nuclear magnetic resonance spectroscopy and infrared spectroscopy suggest that the polymerization was successfully initiated by inositol and confirm the structure of 6-s-PLGA. The molecular weights of a series of 6-s-PLGAs had a ratio corresponding to the molar ratio of raw materials to initiator. Differential scanning calorimetry revealed that the 6-s-PLGA had a low glass transition temperature of 40°C-50°C. The 6-s-PLGA-PTX-NPs were monodispersed with an average diameter of 240.4±6.9 nm in water, which was further confirmed by transmission electron microscopy. The encapsulation efficiency of the 6-s-PLGA-PTX-NPs was higher than that of the L-PLGA-PTX-NPs. In terms of the in vitro release of nanoparticles, paclitaxel (PTX) was released more slowly and more steadily from 6-s-PLGA than from linear poly(lactic-co-glycolic acid). In the cytostatic study, the 6-s-PLGA-PTX-NPs and L-PLGA-PTX-NPs were found to have a similar antiproliferative effect, which indicates durable efficacy due to the slower release of the PTX when loaded in 6-s-PLGA.
Conclusion: The results suggest that 6-s-PLGA may be promising for application in PTX delivery to enhance sustained antiproliferative therapy.
Keywords: PLGA; PTX; antiproliferative therapy; drug delivery; nanoparticles; polymer.
Figures
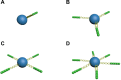
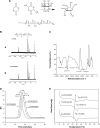
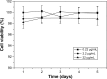
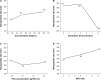
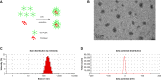
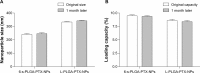
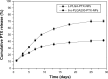
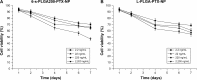
Similar articles
-
Mesenchymal stem cells loaded with paclitaxel-poly(lactic-co-glycolic acid) nanoparticles for glioma-targeting therapy.Int J Nanomedicine. 2018 Sep 7;13:5231-5248. doi: 10.2147/IJN.S167142. eCollection 2018. Int J Nanomedicine. 2018. PMID: 30237710 Free PMC article.
-
Poly-(Lactic-co-Glycolic) Acid Nanoparticles for Synergistic Delivery of Epirubicin and Paclitaxel to Human Lung Cancer Cells.Molecules. 2020 Sep 16;25(18):4243. doi: 10.3390/molecules25184243. Molecules. 2020. PMID: 32947799 Free PMC article.
-
Enhanced in vitro antiproliferative effects of EpCAM antibody-functionalized paclitaxel-loaded PLGA nanoparticles in retinoblastoma cells.Mol Vis. 2011;17:2724-37. Epub 2011 Oct 19. Mol Vis. 2011. Retraction in: Mol Vis. 2013 Jun 06;19:1258. PMID: 22065926 Free PMC article. Retracted.
-
PLGA-based nanoparticles as cancer drug delivery systems.Asian Pac J Cancer Prev. 2014;15(2):517-35. doi: 10.7314/apjcp.2014.15.2.517. Asian Pac J Cancer Prev. 2014. PMID: 24568455 Review.
-
Biomedical applications of PLGA nanoparticles in nanomedicine: advances in drug delivery systems and cancer therapy.Expert Opin Drug Deliv. 2023 Jul-Dec;20(7):937-954. doi: 10.1080/17425247.2023.2223941. Epub 2023 Jun 23. Expert Opin Drug Deliv. 2023. PMID: 37294853 Review.
Cited by
-
The Design of Poly(lactide-co-glycolide) Nanocarriers for Medical Applications.Front Bioeng Biotechnol. 2020 Feb 11;8:48. doi: 10.3389/fbioe.2020.00048. eCollection 2020. Front Bioeng Biotechnol. 2020. PMID: 32117928 Free PMC article. Review.
-
Anti-HER2 PLGA-PEG polymer nanoparticle containing gold nanorods and paclitaxel for laser-activated breast cancer detection and therapy.Biomed Opt Express. 2021 Mar 18;12(4):2171-2185. doi: 10.1364/BOE.419252. eCollection 2021 Apr 1. Biomed Opt Express. 2021. PMID: 33996222 Free PMC article.
-
[Synthesis of amphiphilic block copolymer of PLGA-b-(PEI-co-PEG) and characterization of the self-assembled cationic micelles].Nan Fang Yi Ke Da Xue Xue Bao. 2018 Aug 30;38(9):1139-1146. doi: 10.12122/j.issn.1673-4254.2018.09.20. Nan Fang Yi Ke Da Xue Xue Bao. 2018. PMID: 30377107 Free PMC article. Chinese.
-
Nanoscale cationic micelles of amphiphilic copolymers based on star-shaped PLGA and PEI cross-linked PEG for protein delivery application.J Mater Sci Mater Med. 2019 Aug 7;30(8):93. doi: 10.1007/s10856-019-6294-y. J Mater Sci Mater Med. 2019. PMID: 31392433
-
Synthesis of three-arm block copolymer poly(lactic-co-glycolic acid)-poly(ethylene glycol) with oxalyl chloride and its application in hydrophobic drug delivery.Int J Nanomedicine. 2016 Nov 15;11:6065-6077. doi: 10.2147/IJN.S119446. eCollection 2016. Int J Nanomedicine. 2016. PMID: 27895480 Free PMC article.
References
-
- Zou W, Cao G, Xi Y, Zhang N. New approach for local delivery of rapamycin by bioadhesive PLGA-carbopol nanoparticles. Drug Deliv. 2009;16(1):15–23. - PubMed
-
- Borselli C, Ungaro F, Oliviero O, et al. Bioactivation of collagen matrices through sustained VEGF release from PLGA microspheres. J Biomed Mater Res A. 2010;92(1):94–102. - PubMed
-
- Subramanian S, Pandey U, Gugulothu D, Patravale V, Samuel G. Modification of PLGA Nanoparticles for Improved Properties as a (99m) Tc-Labeled Agent in Sentinel Lymph Node Detection. Cancer Biother Radiopharm. 2013;28(8):598–606. - PubMed
-
- Mittal G, Sahana DK, Bhardwaj V, Ravi Kumar MN. Estradiol loaded PLGA nanoparticles for oral administration: effect of polymer molecular weight and copolymer composition on release behavior in vitro and in vivo. J Control Release. 2007;119(1):77–85. - PubMed
-
- Stanwick JC, Baumann MD, Shoichet MS. Enhanced neurotrophin-3 bioactivity and release from a nanoparticle-loaded composite hydrogel. J Control Release. 2012;160(3):666–675. - PubMed
Publication types
MeSH terms
Substances
LinkOut - more resources
Full Text Sources
Other Literature Sources