Sirtuin 3 (SIRT3) protein regulates long-chain acyl-CoA dehydrogenase by deacetylating conserved lysines near the active site
- PMID: 24121500
- PMCID: PMC3837126
- DOI: 10.1074/jbc.M113.510354
Sirtuin 3 (SIRT3) protein regulates long-chain acyl-CoA dehydrogenase by deacetylating conserved lysines near the active site
Abstract
Long-chain acyl-CoA dehydrogenase (LCAD) is a key mitochondrial fatty acid oxidation enzyme. We previously demonstrated increased LCAD lysine acetylation in SIRT3 knockout mice concomitant with reduced LCAD activity and reduced fatty acid oxidation. To study the effects of acetylation on LCAD and determine sirtuin 3 (SIRT3) target sites, we chemically acetylated recombinant LCAD. Acetylation impeded substrate binding and reduced catalytic efficiency. Deacetylation with recombinant SIRT3 partially restored activity. Residues Lys-318 and Lys-322 were identified as SIRT3-targeted lysines. Arginine substitutions at Lys-318 and Lys-322 prevented the acetylation-induced activity loss. Lys-318 and Lys-322 flank residues Arg-317 and Phe-320, which are conserved among all acyl-CoA dehydrogenases and coordinate the enzyme-bound FAD cofactor in the active site. We propose that acetylation at Lys-318/Lys-322 causes a conformational change which reduces hydride transfer from substrate to FAD. Medium-chain acyl-CoA dehydrogenase and acyl-CoA dehydrogenase 9, two related enzymes with lysines at positions equivalent to Lys-318/Lys-322, were also efficiently deacetylated by SIRT3 following chemical acetylation. These results suggest that acetylation/deacetylation at Lys-318/Lys-322 is a mode of regulating fatty acid oxidation. The same mechanism may regulate other acyl-CoA dehydrogenases.
Keywords: Acyl-CoA Dehydrogenase; Electron Transfer; Enzyme Catalysis; FAD; Fatty Acid Oxidation; Lysine Acetylation; Mitochondria; Posttranslational Modification; Sirtuins.
Figures
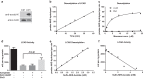
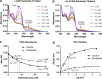
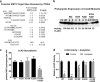
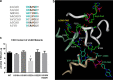
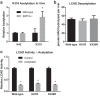
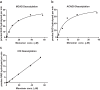
Similar articles
-
SIRT3 and SIRT5 regulate the enzyme activity and cardiolipin binding of very long-chain acyl-CoA dehydrogenase.PLoS One. 2015 Mar 26;10(3):e0122297. doi: 10.1371/journal.pone.0122297. eCollection 2015. PLoS One. 2015. PMID: 25811481 Free PMC article.
-
Obesity-induced lysine acetylation increases cardiac fatty acid oxidation and impairs insulin signalling.Cardiovasc Res. 2014 Sep 1;103(4):485-97. doi: 10.1093/cvr/cvu156. Epub 2014 Jun 25. Cardiovasc Res. 2014. PMID: 24966184 Free PMC article.
-
SIRT3 regulates mitochondrial fatty-acid oxidation by reversible enzyme deacetylation.Nature. 2010 Mar 4;464(7285):121-5. doi: 10.1038/nature08778. Nature. 2010. PMID: 20203611 Free PMC article.
-
Role of CoA and acetyl-CoA in regulating cardiac fatty acid and glucose oxidation.Biochem Soc Trans. 2014 Aug;42(4):1043-51. doi: 10.1042/BST20140094. Biochem Soc Trans. 2014. PMID: 25110000 Review.
-
SIRT3 regulates mitochondrial protein acetylation and intermediary metabolism.Cold Spring Harb Symp Quant Biol. 2011;76:267-77. doi: 10.1101/sqb.2011.76.010850. Epub 2011 Nov 23. Cold Spring Harb Symp Quant Biol. 2011. PMID: 22114326 Review.
Cited by
-
Metabolic reprogramming and epigenetic modifications on the path to cancer.Protein Cell. 2022 Dec;13(12):877-919. doi: 10.1007/s13238-021-00846-7. Epub 2021 May 29. Protein Cell. 2022. PMID: 34050894 Free PMC article. Review.
-
Acetyl-L-carnitine increases mitochondrial protein acetylation in the aged rat heart.Mech Ageing Dev. 2015 Jan;145:39-50. doi: 10.1016/j.mad.2015.01.003. Epub 2015 Feb 7. Mech Ageing Dev. 2015. PMID: 25660059 Free PMC article.
-
SIRT3 a Major Player in Attenuation of Hepatic Ischemia-Reperfusion Injury by Reducing ROS via Its Downstream Mediators: SOD2, CYP-D, and HIF-1α.Oxid Med Cell Longev. 2018 Nov 13;2018:2976957. doi: 10.1155/2018/2976957. eCollection 2018. Oxid Med Cell Longev. 2018. PMID: 30538800 Free PMC article. Review.
-
Divergent serum metabolomic, skeletal muscle signaling, transcriptomic, and performance adaptations to fasted versus whey protein-fed sprint interval training.Am J Physiol Endocrinol Metab. 2021 Dec 1;321(6):E802-E820. doi: 10.1152/ajpendo.00265.2021. Epub 2021 Nov 8. Am J Physiol Endocrinol Metab. 2021. PMID: 34747202 Free PMC article. Clinical Trial.
-
Mitochondrial PKM2 deacetylation by procyanidin B2-induced SIRT3 upregulation alleviates lung ischemia/reperfusion injury.Cell Death Dis. 2022 Jul 11;13(7):594. doi: 10.1038/s41419-022-05051-w. Cell Death Dis. 2022. PMID: 35821123 Free PMC article.
References
-
- He W., Newman J. C., Wang M. Z., Ho L., Verdin E. (2012) Mitochondrial sirtuins. Regulators of protein acylation and metabolism. Trends Endocrinol. Metab. 23, 467–476 - PubMed
Publication types
MeSH terms
Substances
Grants and funding
LinkOut - more resources
Full Text Sources
Other Literature Sources
Molecular Biology Databases