Recruitment of folliculin to lysosomes supports the amino acid-dependent activation of Rag GTPases
- PMID: 24081491
- PMCID: PMC3787382
- DOI: 10.1083/jcb.201307084
Recruitment of folliculin to lysosomes supports the amino acid-dependent activation of Rag GTPases
Abstract
Birt-Hogg-Dubé syndrome, a human disease characterized by fibrofolliculomas (hair follicle tumors) as well as a strong predisposition toward the development of pneumothorax, pulmonary cysts, and renal carcinoma, arises from loss-of-function mutations in the folliculin (FLCN) gene. In this study, we show that FLCN regulates lysosome function by promoting the mTORC1-dependent phosphorylation and cytoplasmic sequestration of transcription factor EB (TFEB). Our results indicate that FLCN is specifically required for the amino acid-stimulated recruitment of mTORC1 to lysosomes by Rag GTPases. We further demonstrated that FLCN itself was selectively recruited to the surface of lysosomes after amino acid depletion and directly bound to RagA via its GTPase domain. FLCN-interacting protein 1 (FNIP1) promotes both the lysosome recruitment and Rag interactions of FLCN. These new findings define the lysosome as a site of action for FLCN and indicate a critical role for FLCN in the amino acid-dependent activation of mTOR via its direct interaction with the RagA/B GTPases.
Figures
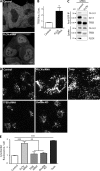
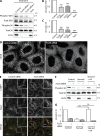
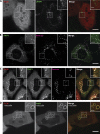
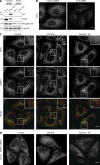
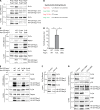
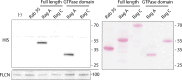
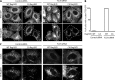
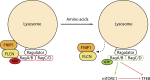
Similar articles
-
Structural mechanism of a Rag GTPase activation checkpoint by the lysosomal folliculin complex.Science. 2019 Nov 22;366(6468):971-977. doi: 10.1126/science.aax0364. Epub 2019 Oct 31. Science. 2019. PMID: 31672913 Free PMC article.
-
The folliculin tumor suppressor is a GAP for the RagC/D GTPases that signal amino acid levels to mTORC1.Mol Cell. 2013 Nov 21;52(4):495-505. doi: 10.1016/j.molcel.2013.09.016. Epub 2013 Oct 3. Mol Cell. 2013. PMID: 24095279 Free PMC article.
-
GATOR1-dependent recruitment of FLCN-FNIP to lysosomes coordinates Rag GTPase heterodimer nucleotide status in response to amino acids.J Cell Biol. 2018 Aug 6;217(8):2765-2776. doi: 10.1083/jcb.201712177. Epub 2018 May 30. J Cell Biol. 2018. PMID: 29848618 Free PMC article.
-
Birt-Hogg-Dubé syndrome: Clinical and molecular aspects of recently identified kidney cancer syndrome.Int J Urol. 2016 Mar;23(3):204-10. doi: 10.1111/iju.13015. Epub 2015 Nov 25. Int J Urol. 2016. PMID: 26608100 Review.
-
FLCN: The causative gene for Birt-Hogg-Dubé syndrome.Gene. 2018 Jan 15;640:28-42. doi: 10.1016/j.gene.2017.09.044. Epub 2017 Sep 29. Gene. 2018. PMID: 28970150 Free PMC article. Review.
Cited by
-
Transcriptional and epigenetic regulation of autophagy in aging.Autophagy. 2015;11(6):867-80. doi: 10.1080/15548627.2015.1034410. Autophagy. 2015. PMID: 25836756 Free PMC article. Review.
-
The progress in C9orf72 research: ALS/FTD pathogenesis, functions and structure.Small GTPases. 2022 Jan;13(1):56-76. doi: 10.1080/21541248.2021.1892443. Epub 2021 Mar 5. Small GTPases. 2022. PMID: 33663328 Free PMC article. Review.
-
PRDM10 directs FLCN expression in a novel disorder overlapping with Birt-Hogg-Dubé syndrome and familial lipomatosis.Hum Mol Genet. 2023 Mar 20;32(7):1223-1235. doi: 10.1093/hmg/ddac288. Hum Mol Genet. 2023. PMID: 36440963 Free PMC article.
-
An mTORC1-mediated negative feedback loop constrains amino acid-induced FLCN-Rag activation in renal cells with TSC2 loss.Nat Commun. 2022 Nov 10;13(1):6808. doi: 10.1038/s41467-022-34617-7. Nat Commun. 2022. PMID: 36357396 Free PMC article.
-
Nutrients: Signal 4 in T cell immunity.J Exp Med. 2024 Mar 4;221(3):e20221839. doi: 10.1084/jem.20221839. Epub 2024 Feb 27. J Exp Med. 2024. PMID: 38411744 Free PMC article.
References
-
- Baba M., Hong S.B., Sharma N., Warren M.B., Nickerson M.L., Iwamatsu A., Esposito D., Gillette W.K., Hopkins R.F., III, Hartley J.L., et al. 2006. Folliculin encoded by the BHD gene interacts with a binding protein, FNIP1, and AMPK, and is involved in AMPK and mTOR signaling. Proc. Natl. Acad. Sci. USA. 103:15552–15557 10.1073/pnas.0603781103 - DOI - PMC - PubMed
-
- Bar-Peled L., Chantranupong L., Cherniack A.D., Chen W.W., Ottina K.A., Grabiner B.C., Spear E.D., Carter S.L., Meyerson M., Sabatini D.M. 2013. A Tumor suppressor complex with GAP activity for the Rag GTPases that signal amino acid sufficiency to mTORC1. Science. 340:1100–1106 10.1126/science.1232044 - DOI - PMC - PubMed
Publication types
MeSH terms
Substances
Grants and funding
LinkOut - more resources
Full Text Sources
Other Literature Sources
Molecular Biology Databases
Research Materials
Miscellaneous