Mitochondrial dynamics controlled by mitofusins regulate Agrp neuronal activity and diet-induced obesity
- PMID: 24074868
- PMCID: PMC4142434
- DOI: 10.1016/j.cell.2013.09.004
Mitochondrial dynamics controlled by mitofusins regulate Agrp neuronal activity and diet-induced obesity
Abstract
Mitochondria are key organelles in the maintenance of cellular energy metabolism and integrity. Here, we show that mitochondria number decrease but their size increase in orexigenic agouti-related protein (Agrp) neurons during the transition from fasted to fed to overfed state. These fusion-like dynamic changes were cell-type specific, as they occurred in the opposite direction in anorexigenic pro-opiomelanocortin (POMC) neurons. Interfering with mitochondrial fusion mechanisms in Agrp neurons by cell-selectively knocking down mitofusin 1 (Mfn1) or mitofusin 2 (Mfn2) resulted in altered mitochondria size and density in these cells. Deficiency in mitofusins impaired the electric activity of Agrp neurons during high-fat diet (HFD), an event reversed by cell-selective administration of ATP. Agrp-specific Mfn1 or Mfn2 knockout mice gained less weight when fed a HFD due to decreased fat mass. Overall, our data unmask an important role for mitochondrial dynamics governed by Mfn1 and Mfn2 in Agrp neurons in central regulation of whole-body energy metabolism.
Copyright © 2013 Elsevier Inc. All rights reserved.
Figures
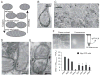
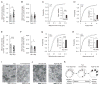
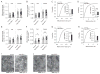
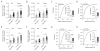
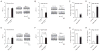
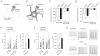
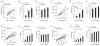
Comment in
-
Mitofusins: mighty regulators of metabolism.Cell. 2013 Sep 26;155(1):17-8. doi: 10.1016/j.cell.2013.09.013. Cell. 2013. PMID: 24074856
-
Neuroendocrinology: Mitofusins and energy balance.Nat Rev Endocrinol. 2013 Dec;9(12):691. doi: 10.1038/nrendo.2013.202. Epub 2013 Oct 15. Nat Rev Endocrinol. 2013. PMID: 24126482 No abstract available.
Similar articles
-
Suppression of GHS-R in AgRP Neurons Mitigates Diet-Induced Obesity by Activating Thermogenesis.Int J Mol Sci. 2017 Apr 14;18(4):832. doi: 10.3390/ijms18040832. Int J Mol Sci. 2017. PMID: 28420089 Free PMC article.
-
AgRP Neuron-Specific Deletion of Glucocorticoid Receptor Leads to Increased Energy Expenditure and Decreased Body Weight in Female Mice on a High-Fat Diet.Endocrinology. 2016 Apr;157(4):1457-66. doi: 10.1210/en.2015-1430. Epub 2016 Feb 18. Endocrinology. 2016. PMID: 26889940
-
Targeted Deletion of Mitofusin 1 and Mitofusin 2 Causes Female Infertility and Loss of Follicular Reserve.Reprod Sci. 2023 Feb;30(2):560-568. doi: 10.1007/s43032-022-01014-w. Epub 2022 Jun 23. Reprod Sci. 2023. PMID: 35739352
-
Structure, function, and regulation of mitofusin-2 in health and disease.Biol Rev Camb Philos Soc. 2018 May;93(2):933-949. doi: 10.1111/brv.12378. Epub 2017 Oct 25. Biol Rev Camb Philos Soc. 2018. PMID: 29068134 Free PMC article. Review.
-
Targeting AgRP neurons to maintain energy balance: Lessons from animal models.Biochem Pharmacol. 2018 Sep;155:224-232. doi: 10.1016/j.bcp.2018.07.008. Epub 2018 Jul 18. Biochem Pharmacol. 2018. PMID: 30012460 Review.
Cited by
-
Estrogen-related receptor α (ERRα) and ERRγ are essential coordinators of cardiac metabolism and function.Mol Cell Biol. 2015 Apr;35(7):1281-98. doi: 10.1128/MCB.01156-14. Epub 2015 Jan 26. Mol Cell Biol. 2015. PMID: 25624346 Free PMC article.
-
Hypothalamic innate immune reaction in obesity.Nat Rev Endocrinol. 2015 Jun;11(6):339-51. doi: 10.1038/nrendo.2015.48. Epub 2015 Mar 31. Nat Rev Endocrinol. 2015. PMID: 25824676 Review.
-
Melatonin Improves Mitochondrial Dynamics and Function in the Kidney of Zücker Diabetic Fatty Rats.J Clin Med. 2020 Sep 10;9(9):2916. doi: 10.3390/jcm9092916. J Clin Med. 2020. PMID: 32927647 Free PMC article.
-
The social nature of mitochondria: Implications for human health.Neurosci Biobehav Rev. 2021 Jan;120:595-610. doi: 10.1016/j.neubiorev.2020.04.017. Epub 2020 Jul 8. Neurosci Biobehav Rev. 2021. PMID: 32651001 Free PMC article. Review.
-
Hypothalamic Mitochondrial Dysfunction as a Target in Obesity and Metabolic Disease.Front Endocrinol (Lausanne). 2018 May 31;9:283. doi: 10.3389/fendo.2018.00283. eCollection 2018. Front Endocrinol (Lausanne). 2018. PMID: 29904371 Free PMC article. Review.
References
-
- Attwell D, Laughlin SB. An energy budget for signaling in the grey matter of the brain. Journal of cerebral blood flow and metabolism: official journal of the International Society of Cerebral Blood Flow and Metabolism. 2001;21:1133–1145. - PubMed
-
- Bach D, Pich S, Soriano FX, Vega N, Baumgartner B, Oriola J, Daugaard JR, Lloberas J, Camps M, Zierath JR, et al. Mitofusin-2 determines mitochondrial network architecture and mitochondrial metabolism. A novel regulatory mechanism altered in obesity. The Journal of biological chemistry. 2003;278:17190–17197. - PubMed
-
- Broberger C, Johansen J, Johansson C, Schalling M, Hokfelt T. The neuropeptide Y agouti gene-related protein (AGRP) brain circuitry in normal, anorectic, and monosodium glutamate-treated mice. Proceedings of the National Academy of Sciences of the United States of America. 1998;95:15043–15048. - PMC - PubMed
Publication types
MeSH terms
Substances
Grants and funding
LinkOut - more resources
Full Text Sources
Other Literature Sources
Medical
Molecular Biology Databases
Miscellaneous