Integrative approaches for finding modular structure in biological networks
- PMID: 24045689
- PMCID: PMC3940161
- DOI: 10.1038/nrg3552
Integrative approaches for finding modular structure in biological networks
Abstract
A central goal of systems biology is to elucidate the structural and functional architecture of the cell. To this end, large and complex networks of molecular interactions are being rapidly generated for humans and model organisms. A recent focus of bioinformatics research has been to integrate these networks with each other and with diverse molecular profiles to identify sets of molecules and interactions that participate in a common biological function - that is, 'modules'. Here, we classify such integrative approaches into four broad categories, describe their bioinformatic principles and review their applications.
Figures
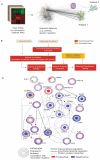
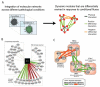
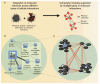
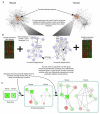
Similar articles
-
Probabilistic integrative modeling of genome-scale metabolic and regulatory networks in Escherichia coli and Mycobacterium tuberculosis.Proc Natl Acad Sci U S A. 2010 Oct 12;107(41):17845-50. doi: 10.1073/pnas.1005139107. Epub 2010 Sep 27. Proc Natl Acad Sci U S A. 2010. PMID: 20876091 Free PMC article.
-
A genome-scale, constraint-based approach to systems biology of human metabolism.Mol Biosyst. 2007 Sep;3(9):598-603. doi: 10.1039/b705597h. Epub 2007 Jul 11. Mol Biosyst. 2007. PMID: 17700859
-
A Protocol for the Construction and Curation of Genome-Scale Integrated Metabolic and Regulatory Network Models.Methods Mol Biol. 2019;1927:203-214. doi: 10.1007/978-1-4939-9142-6_14. Methods Mol Biol. 2019. PMID: 30788794
-
Modular analysis of biological networks.Adv Exp Med Biol. 2012;736:3-17. doi: 10.1007/978-1-4419-7210-1_1. Adv Exp Med Biol. 2012. PMID: 22161320 Review.
-
Bacterial molecular networks: bridging the gap between functional genomics and dynamical modelling.Methods Mol Biol. 2012;804:1-11. doi: 10.1007/978-1-61779-361-5_1. Methods Mol Biol. 2012. PMID: 22144145 Review.
Cited by
-
NetworkAnalyst for statistical, visual and network-based meta-analysis of gene expression data.Nat Protoc. 2015 Jun;10(6):823-44. doi: 10.1038/nprot.2015.052. Epub 2015 May 7. Nat Protoc. 2015. PMID: 25950236
-
Genetic variants in Alzheimer disease - molecular and brain network approaches.Nat Rev Neurol. 2016 Jul;12(7):413-27. doi: 10.1038/nrneurol.2016.84. Epub 2016 Jun 10. Nat Rev Neurol. 2016. PMID: 27282653 Free PMC article. Review.
-
Pathway-based protein-protein association network to explore mechanism of α-glucosidase inhibitors from Scutellaria baicalensis Georgi against type 2 diabetes.IET Syst Biol. 2021 Jun;15(4):126-135. doi: 10.1049/syb2.12019. Epub 2021 Apr 26. IET Syst Biol. 2021. PMID: 33900023 Free PMC article.
-
NetMix2: A Principled Network Propagation Algorithm for Identifying Altered Subnetworks.J Comput Biol. 2022 Dec;29(12):1305-1323. doi: 10.1089/cmb.2022.0336. J Comput Biol. 2022. PMID: 36525308 Free PMC article.
-
Identifying significantly impacted pathways: a comprehensive review and assessment.Genome Biol. 2019 Oct 9;20(1):203. doi: 10.1186/s13059-019-1790-4. Genome Biol. 2019. PMID: 31597578 Free PMC article. Review.
References
-
- Hartwell LH, Hopfield JJ, Leibler S, Murray AW. From molecular to modular cell biology. Nature. 1999;402:C47–52. - PubMed
-
- Alon U. Biological networks: the tinkerer as an engineer. Science. 2003;301:1866–7. - PubMed
-
- Stuart JM, Segal E, Koller D, Kim SK. A gene-coexpression network for global discovery of conserved genetic modules. Science. 2003;302:249–55. - PubMed
-
- Segal E, et al. Module networks: identifying regulatory modules and their condition-specific regulators from gene expression data. Nat Genet. 2003;34:166–76. - PubMed
Publication types
MeSH terms
Grants and funding
LinkOut - more resources
Full Text Sources
Other Literature Sources