An integrin-linked machinery of cytoskeletal regulation that enables experimental tumor initiation and metastatic colonization
- PMID: 24035453
- PMCID: PMC3864118
- DOI: 10.1016/j.ccr.2013.08.012
An integrin-linked machinery of cytoskeletal regulation that enables experimental tumor initiation and metastatic colonization
Erratum in
- Cancer Cell. 2013 Dec 9;24(6):806-8
Abstract
Recently extravasated metastatic cancer cells use the Rif/mDia2 actin-nucleating/polymerizing machinery in order to extend integrin β1-containing, filopodium-like protrusions (FLPs), which enable them to interact productively with the surrounding extracellular matrix; this process governs the initial proliferation of these cancer cells. Here, we identify the signaling pathway governing FLP lifetime, which involves integrin-linked kinase (ILK) and β-parvin, two integrin:actin-bridging proteins that block cofilin-mediated actin-filament severing. Notably, the combined actions of Rif/mDia2 and ILK/β-parvin/cofilin pathways on FLPs are required not only for metastatic outgrowth but also for primary tumor formation following experimental implantation. This provides one mechanistic explanation for how the epithelial-mesenchymal transition (EMT) program imparts tumor-initiating powers to carcinoma cells, since it enhances FLP formation through the activation of ILK/β-parvin/cofilin pathway.
Copyright © 2013 Elsevier Inc. All rights reserved.
Figures
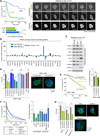
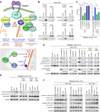
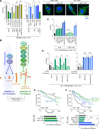
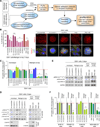
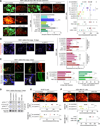
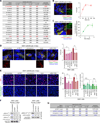
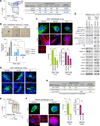
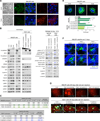
Similar articles
-
Molecular dissection of the ILK-PINCH-parvin triad reveals a fundamental role for the ILK kinase domain in the late stages of focal-adhesion maturation.J Cell Sci. 2009 Jun 1;122(Pt 11):1800-11. doi: 10.1242/jcs.044602. Epub 2009 May 12. J Cell Sci. 2009. PMID: 19435803
-
Involvement of β1-integrin via PIP complex and FAK/paxillin in dexamethasone-induced human mesenchymal stem cells migration.J Cell Physiol. 2011 Mar;226(3):683-92. doi: 10.1002/jcp.22383. J Cell Physiol. 2011. PMID: 20717960
-
The outgrowth of micrometastases is enabled by the formation of filopodium-like protrusions.Cancer Discov. 2012 Aug;2(8):706-21. doi: 10.1158/2159-8290.CD-11-0239. Epub 2012 May 18. Cancer Discov. 2012. PMID: 22609699 Free PMC article.
-
The integrin-actin connection, an eternal love affair.EMBO J. 2003 May 15;22(10):2324-33. doi: 10.1093/emboj/cdg245. EMBO J. 2003. PMID: 12743027 Free PMC article. Review.
-
ILK, PINCH and parvin: the tIPP of integrin signalling.Nat Rev Mol Cell Biol. 2006 Jan;7(1):20-31. doi: 10.1038/nrm1789. Nat Rev Mol Cell Biol. 2006. PMID: 16493410 Review.
Cited by
-
Mechanical Cues Affect Migration and Invasion of Cells From Three Different Directions.Front Cell Dev Biol. 2020 Sep 17;8:583226. doi: 10.3389/fcell.2020.583226. eCollection 2020. Front Cell Dev Biol. 2020. PMID: 33043017 Free PMC article. Review.
-
Epithelial morphogenesis in the Drosophila egg chamber requires Parvin and ILK.Front Cell Dev Biol. 2022 Dec 1;10:951082. doi: 10.3389/fcell.2022.951082. eCollection 2022. Front Cell Dev Biol. 2022. PMID: 36531940 Free PMC article.
-
TEAD4 functions as a prognostic biomarker and triggers EMT via PI3K/AKT pathway in bladder cancer.J Exp Clin Cancer Res. 2022 May 17;41(1):175. doi: 10.1186/s13046-022-02377-3. J Exp Clin Cancer Res. 2022. PMID: 35581606 Free PMC article.
-
ILK promotes angiogenic activity of mesenchymal stem cells in multiple myeloma.Oncol Lett. 2018 Jul;16(1):1101-1106. doi: 10.3892/ol.2018.8711. Epub 2018 May 16. Oncol Lett. 2018. PMID: 29963187 Free PMC article.
-
Technical Advancements for Studying Immune Regulation of Disseminated Dormant Cancer Cells.Front Oncol. 2020 Nov 4;10:594514. doi: 10.3389/fonc.2020.594514. eCollection 2020. Front Oncol. 2020. PMID: 33251149 Free PMC article. Review.
References
-
- Bokoch GM. Biology of the p21-activated kinases. Annu Rev Biochem. 2003;72:743–781. - PubMed
-
- Chambers AF, Groom AC, MacDonald IC. Dissemination and growth of cancer cells in metastatic sites. Nat Rev Cancer. 2002;2:563–572. - PubMed
-
- Clevers H. The cancer stem cell: premises, promises and challenges. Nat Med. 2011;17:313–319. - PubMed
Publication types
MeSH terms
Substances
Grants and funding
LinkOut - more resources
Full Text Sources
Other Literature Sources
Molecular Biology Databases