The effect of heart rate on the heart rate variability response to autonomic interventions
- PMID: 23986716
- PMCID: PMC3752439
- DOI: 10.3389/fphys.2013.00222
The effect of heart rate on the heart rate variability response to autonomic interventions
Abstract
Heart rate variability (HRV), the beat-to-beat variation in either heart rate (HR) or heart period (R-R interval), has become a popular clinical and investigational tool to quantify cardiac autonomic regulation. However, it is not widely appreciated that, due to the inverse curvilinear relationship between HR and R-R interval, HR per se can profoundly influence HRV. It is, therefore, critical to correct HRV for the prevailing HR particularly, as HR changes in response to autonomic neural activation or inhibition. The present study evaluated the effects of HR on the HRV response to autonomic interventions that either increased (submaximal exercise, n = 25 or baroreceptor reflex activation, n = 20) or reduced (pharmacological blockade: β-adrenergic receptor, muscarinic receptor antagonists alone and in combination, n = 25, or bilateral cervical vagotomy, n = 9) autonomic neural activity in a canine model. Both total (RR interval standard deviation, RRSD) and the high frequency (HF) variability (HF, 0.24-1.04 Hz) were determined before and in response to an autonomic intervention. All interventions that reduced or abolished cardiac parasympathetic regulation provoked large reductions in HRV even after HR correction [division by mean RRsec or (mean RRsec)(2) for RRSD and HF, respectively] while interventions that reduced HR yielded mixed results. β-adrenergic receptor blockade reduced HRV (RRSD but not HF) while both RRSD and HF increased in response to increases in arterial blood (baroreceptor reflex activation) even after HR correction. These data suggest that the physiological basis for HRV is revealed after correction for prevailing HR and, further, that cardiac parasympathetic activity is responsible for a major portion of the HRV in the dog.
Keywords: autonomic nervous system; baroreceptor reflex; cholinergic receptor antagonists; exercise; heart rate; heart rate variability; β-adrenergic receptors.
Figures
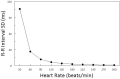
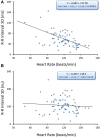
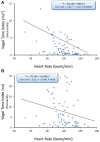
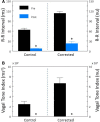
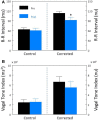
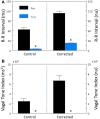
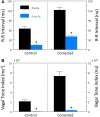
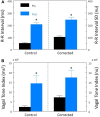
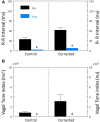
Similar articles
-
Autonomic effects on the spectral analysis of heart rate variability after exercise.Am J Physiol Heart Circ Physiol. 2009 Oct;297(4):H1421-8. doi: 10.1152/ajpheart.00217.2009. Epub 2009 Jul 31. Am J Physiol Heart Circ Physiol. 2009. PMID: 19648255 Free PMC article.
-
Exercise training-induced bradycardia: evidence for enhanced parasympathetic regulation without changes in intrinsic sinoatrial node function.J Appl Physiol (1985). 2015 Jun 1;118(11):1344-55. doi: 10.1152/japplphysiol.01111.2014. Epub 2015 Mar 6. J Appl Physiol (1985). 2015. PMID: 25749448 Free PMC article.
-
Changes in Heart Rate and Its Regulation by the Autonomic Nervous System Do Not Differ Between Forced and Voluntary Exercise in Mice.Front Physiol. 2018 Jul 16;9:841. doi: 10.3389/fphys.2018.00841. eCollection 2018. Front Physiol. 2018. PMID: 30061838 Free PMC article.
-
Monitoring Athletic Training Status Through Autonomic Heart Rate Regulation: A Systematic Review and Meta-Analysis.Sports Med. 2016 Oct;46(10):1461-86. doi: 10.1007/s40279-016-0484-2. Sports Med. 2016. PMID: 26888648 Review.
-
Autonomic dysfunction and heart rate variability with Holter monitoring: a diagnostic look at autonomic regulation.Herzschrittmacherther Elektrophysiol. 2021 Sep;32(3):315-319. doi: 10.1007/s00399-021-00780-5. Epub 2021 Jul 8. Herzschrittmacherther Elektrophysiol. 2021. PMID: 34236476 Review. English.
Cited by
-
The Relationship between Expressive/Suppressive Hostility Behavior and Cardiac Autonomic Activations in Patients with Coronary Artery Disease.Acta Cardiol Sin. 2015 Jul;31(4):308-16. doi: 10.6515/acs20141027b. Acta Cardiol Sin. 2015. PMID: 27122887 Free PMC article.
-
Comparison of Autonomic Reactions during Urodynamic Examination in Patients with Spinal Cord Injuries and Able-Bodied Subjects.PLoS One. 2016 Aug 30;11(8):e0161976. doi: 10.1371/journal.pone.0161976. eCollection 2016. PLoS One. 2016. PMID: 27575616 Free PMC article.
-
The impact of stress and anesthesia on animal models of infectious disease.Front Vet Sci. 2023 Feb 2;10:1086003. doi: 10.3389/fvets.2023.1086003. eCollection 2023. Front Vet Sci. 2023. PMID: 36816193 Free PMC article. Review.
-
Effect of a lactate-guided conditioning program on heart rate variability obtained using 24-Holter electrocardiography in Beagle dogs.PLoS One. 2020 Jun 1;15(6):e0233264. doi: 10.1371/journal.pone.0233264. eCollection 2020. PLoS One. 2020. PMID: 32479554 Free PMC article.
-
Functional autonomic nervous system profile in children with autism spectrum disorder.Mol Autism. 2014 Jul 4;5:39. doi: 10.1186/2040-2392-5-39. eCollection 2014. Mol Autism. 2014. PMID: 25031832 Free PMC article.
References
LinkOut - more resources
Full Text Sources
Other Literature Sources
Research Materials
Miscellaneous