Dissecting the interactions of SERRATE with RNA and DICER-LIKE 1 in Arabidopsis microRNA precursor processing
- PMID: 23921632
- PMCID: PMC3799435
- DOI: 10.1093/nar/gkt667
Dissecting the interactions of SERRATE with RNA and DICER-LIKE 1 in Arabidopsis microRNA precursor processing
Abstract
Efficient and precise microRNA (miRNA) biogenesis in Arabidopsis is mediated by the RNaseIII-family enzyme DICER-LIKE 1 (DCL1), double-stranded RNA-binding protein HYPONASTIC LEAVES 1 and the zinc-finger (ZnF) domain-containing protein SERRATE (SE). In the present study, we examined primary miRNA precursor (pri-miRNA) processing by highly purified recombinant DCL1 and SE proteins and found that SE is integral to pri-miRNA processing by DCL1. SE stimulates DCL1 cleavage of the pri-miRNA in an ionic strength-dependent manner. SE uses its N-terminal domain to bind to RNA and requires both N-terminal and ZnF domains to bind to DCL1. However, when DCL1 is bound to RNA, the interaction with the ZnF domain of SE becomes indispensible and stimulates the activity of DCL1 without requiring SE binding to RNA. Our results suggest that the interactions among SE, DCL1 and RNA are a potential point for regulating pri-miRNA processing.
Figures
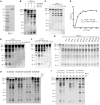
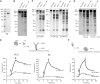
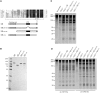
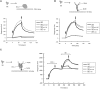
Similar articles
-
Molecular insights into miRNA processing by Arabidopsis thaliana SERRATE.Nucleic Acids Res. 2011 Sep 1;39(17):7828-36. doi: 10.1093/nar/gkr428. Epub 2011 Jun 17. Nucleic Acids Res. 2011. PMID: 21685453 Free PMC article.
-
The RNA-binding proteins HYL1 and SE promote accurate in vitro processing of pri-miRNA by DCL1.Proc Natl Acad Sci U S A. 2008 Jul 22;105(29):9970-5. doi: 10.1073/pnas.0803356105. Epub 2008 Jul 16. Proc Natl Acad Sci U S A. 2008. PMID: 18632569 Free PMC article.
-
Homodimerization of HYL1 ensures the correct selection of cleavage sites in primary miRNA.Nucleic Acids Res. 2014 Oct 29;42(19):12224-36. doi: 10.1093/nar/gku907. Epub 2014 Oct 7. Nucleic Acids Res. 2014. PMID: 25294831 Free PMC article.
-
SERRATE: a key factor in coordinated RNA processing in plants.Trends Plant Sci. 2023 Jul;28(7):841-853. doi: 10.1016/j.tplants.2023.03.009. Epub 2023 Apr 4. Trends Plant Sci. 2023. PMID: 37019716 Review.
-
New insights into pri-miRNA processing and accumulation in plants.Wiley Interdiscip Rev RNA. 2015 Sep-Oct;6(5):533-45. doi: 10.1002/wrna.1292. Epub 2015 Jun 29. Wiley Interdiscip Rev RNA. 2015. PMID: 26119101 Review.
Cited by
-
Plant dicer-like proteins: double-stranded RNA-cleaving enzymes for small RNA biogenesis.J Plant Res. 2017 Jan;130(1):33-44. doi: 10.1007/s10265-016-0877-1. Epub 2016 Nov 24. J Plant Res. 2017. PMID: 27885504 Review.
-
microRNA biogenesis and stabilization in plants.Fundam Res. 2023 Mar 17;3(5):707-717. doi: 10.1016/j.fmre.2023.02.023. eCollection 2023 Sep. Fundam Res. 2023. PMID: 38933298 Free PMC article. Review.
-
Biochemical requirements for two Dicer-like activities from wheat germ.PLoS One. 2015 Jan 23;10(1):e0116736. doi: 10.1371/journal.pone.0116736. eCollection 2015. PLoS One. 2015. PMID: 25615604 Free PMC article.
-
PRP4KA phosphorylates SERRATE for degradation via 20S proteasome to fine-tune miRNA production in Arabidopsis.Sci Adv. 2022 Mar 25;8(12):eabm8435. doi: 10.1126/sciadv.abm8435. Epub 2022 Mar 25. Sci Adv. 2022. PMID: 35333566 Free PMC article.
-
Regulation of embryonic and adult neurogenesis by Ars2.Development. 2020 Jan 22;147(2):dev180018. doi: 10.1242/dev.180018. Development. 2020. PMID: 31969356 Free PMC article.
References
-
- Voinnet O. Origin, biogenesis, and activity of plant microRNAs. Cell. 2009;136:669–687. - PubMed
-
- Kim VN, Han J, Siomi MC. Biogenesis of small RNAs in animals. Nat Rev Mol Cell Biol. 2009;10:126–139. - PubMed
-
- Yang L, Liu Z, Lu F, Dong A, Huang H. SERRATE is a novel nuclear regulator in primary microRNA processing in Arabidopsis. Plant J. 2006;47:841–850. - PubMed
Publication types
MeSH terms
Substances
LinkOut - more resources
Full Text Sources
Other Literature Sources
Molecular Biology Databases