ATPase-driven oligomerization of RIG-I on RNA allows optimal activation of type-I interferon
- PMID: 23846310
- PMCID: PMC3790048
- DOI: 10.1038/embor.2013.102
ATPase-driven oligomerization of RIG-I on RNA allows optimal activation of type-I interferon
Abstract
The cytosolic pathogen sensor RIG-I is activated by RNAs with exposed 5'-triphosphate (5'-ppp) and terminal double-stranded structures, such as those that are generated during viral infection. RIG-I has been shown to translocate on dsRNA in an ATP-dependent manner. However, the precise role of the ATPase activity in RIG-I activation remains unclear. Using in vitro-transcribed Sendai virus defective interfering RNA as a model ligand, we show that RIG-I oligomerizes on 5'-ppp dsRNA in an ATP hydrolysis-dependent and dsRNA length-dependent manner, which correlates with the strength of type-I interferon (IFN-I) activation. These results establish a clear role for the ligand-induced ATPase activity of RIG-I in the stimulation of the IFN response.
Conflict of interest statement
The authors declare that they have no conflict of interest.
Figures
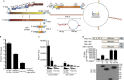
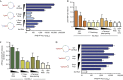
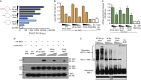
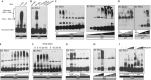
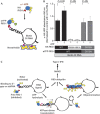
Comment in
-
RNA sensing: the more RIG-I the merrier?EMBO Rep. 2013 Sep;14(9):751-2. doi: 10.1038/embor.2013.120. Epub 2013 Aug 6. EMBO Rep. 2013. PMID: 23917614 Free PMC article. No abstract available.
Similar articles
-
RIG-I ATPase activity and discrimination of self-RNA versus non-self-RNA.mBio. 2015 Mar 3;6(2):e02349. doi: 10.1128/mBio.02349-14. mBio. 2015. PMID: 25736886 Free PMC article.
-
Differential recognition of viral RNA by RIG-I.Virulence. 2011 Mar-Apr;2(2):166-9. doi: 10.4161/viru.2.2.15481. Epub 2011 Mar 1. Virulence. 2011. PMID: 21422808 Free PMC article.
-
ATP hydrolysis by the viral RNA sensor RIG-I prevents unintentional recognition of self-RNA.Elife. 2015 Nov 26;4:e10859. doi: 10.7554/eLife.10859. Elife. 2015. PMID: 26609812 Free PMC article.
-
Sensing of viral nucleic acids by RIG-I: from translocation to translation.Eur J Cell Biol. 2012 Jan;91(1):78-85. doi: 10.1016/j.ejcb.2011.01.015. Epub 2011 Apr 14. Eur J Cell Biol. 2012. PMID: 21496944 Free PMC article. Review.
-
Links between recognition and degradation of cytoplasmic viral RNA in innate immune response.Rev Med Virol. 2016 Mar;26(2):90-101. doi: 10.1002/rmv.1865. Epub 2015 Dec 8. Rev Med Virol. 2016. PMID: 26643446 Review.
Cited by
-
MDA5-filament, dynamics and disease.Curr Opin Virol. 2015 Jun;12:20-5. doi: 10.1016/j.coviro.2015.01.011. Epub 2015 Feb 9. Curr Opin Virol. 2015. PMID: 25676875 Free PMC article. Review.
-
Multilayered regulations of RIG-I in the anti-viral signaling pathway.J Microbiol. 2016 Sep;54(9):583-587. doi: 10.1007/s12275-016-6322-2. Epub 2016 Aug 31. J Microbiol. 2016. PMID: 27572506 Review.
-
RNA sensing: the more RIG-I the merrier?EMBO Rep. 2013 Sep;14(9):751-2. doi: 10.1038/embor.2013.120. Epub 2013 Aug 6. EMBO Rep. 2013. PMID: 23917614 Free PMC article. No abstract available.
-
Comparative Structure and Function Analysis of the RIG-I-Like Receptors: RIG-I and MDA5.Front Immunol. 2019 Jul 17;10:1586. doi: 10.3389/fimmu.2019.01586. eCollection 2019. Front Immunol. 2019. PMID: 31379819 Free PMC article. Review.
-
Paramyxovirus V Proteins Interact with the RIG-I/TRIM25 Regulatory Complex and Inhibit RIG-I Signaling.J Virol. 2018 Feb 26;92(6):e01960-17. doi: 10.1128/JVI.01960-17. Print 2018 Mar 15. J Virol. 2018. PMID: 29321315 Free PMC article.
References
-
- Ranjan P, Bowzard JB, Schwerzmann JW, Jeisy-Scott V, Fujita T, Sambhara S (2009) Cytoplasmic nucleic acid sensors in antiviral immunity. Trends Mol Med 15: 359–368 - PubMed
-
- Yoneyama M, Kikuchi M, Natsukawa T, Shinobu N, Imaizumi T, Miyagishi M, Taira K, Akira S, Fujita T (2004) The RNA helicase RIG-I has an essential function in double-stranded RNA-induced innate antiviral responses. Nat Immunol 5: 730–737 - PubMed
Publication types
MeSH terms
Substances
Grants and funding
LinkOut - more resources
Full Text Sources
Other Literature Sources