Increased acid stability of the hemagglutinin protein enhances H5N1 influenza virus growth in the upper respiratory tract but is insufficient for transmission in ferrets
- PMID: 23824818
- PMCID: PMC3754100
- DOI: 10.1128/JVI.01175-13
Increased acid stability of the hemagglutinin protein enhances H5N1 influenza virus growth in the upper respiratory tract but is insufficient for transmission in ferrets
Abstract
Influenza virus entry is mediated by the acidic-pH-induced activation of hemagglutinin (HA) protein. Here, we investigated how a decrease in the HA activation pH (an increase in acid stability) influences the properties of highly pathogenic H5N1 influenza virus in mammalian hosts. We generated isogenic A/Vietnam/1203/2004 (H5N1) (VN1203) viruses containing either wild-type HA protein (activation pH 6.0) or an HA2-K58I point mutation (K to I at position 58) (activation pH 5.5). The VN1203-HA2-K58I virus had replication kinetics similar to those of wild-type VN1203 in MDCK and normal human bronchial epithelial cells and yet had reduced growth in human alveolar A549 cells, which were found to have a higher endosomal pH than MDCK cells. Wild-type and HA2-K58I viruses promoted similar levels of morbidity and mortality in C57BL/6J mice and ferrets, and neither virus transmitted efficiently to naive contact cage-mate ferrets. The acid-stabilizing HA2-K58I mutation, which diminishes H5N1 replication and transmission in ducks, increased the virus load in the ferret nasal cavity early during infection while simultaneously reducing the virus load in the lungs. Overall, a single, acid-stabilizing mutation was found to enhance the growth of an H5N1 influenza virus in the mammalian upper respiratory tract, and yet it was insufficient to enable contact transmission in ferrets in the absence of additional mutations that confer α(2,6) receptor binding specificity and remove a critical N-linked glycosylation site. The information provided here on the contribution of HA acid stability to H5N1 influenza virus fitness and transmissibility in mammals in the background of a non-laboratory-adapted virus provides essential information for the surveillance and assessment of the pandemic potential of currently circulating H5N1 viruses.
Figures
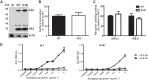
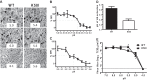
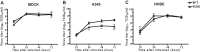
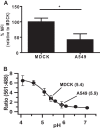
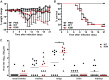
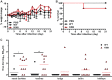
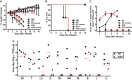
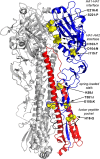
Similar articles
-
Mutations in haemagglutinin that affect receptor binding and pH stability increase replication of a PR8 influenza virus with H5 HA in the upper respiratory tract of ferrets and may contribute to transmissibility.J Gen Virol. 2013 Jun;94(Pt 6):1220-1229. doi: 10.1099/vir.0.050526-0. Epub 2013 Mar 13. J Gen Virol. 2013. PMID: 23486663 Free PMC article.
-
Experimental adaptation of an influenza H5 HA confers respiratory droplet transmission to a reassortant H5 HA/H1N1 virus in ferrets.Nature. 2012 May 2;486(7403):420-8. doi: 10.1038/nature10831. Nature. 2012. PMID: 22722205 Free PMC article.
-
HA-Dependent Tropism of H5N1 and H7N9 Influenza Viruses to Human Endothelial Cells Is Determined by Reduced Stability of the HA, Which Allows the Virus To Cope with Inefficient Endosomal Acidification and Constitutively Expressed IFITM3.J Virol. 2019 Dec 12;94(1):e01223-19. doi: 10.1128/JVI.01223-19. Print 2019 Dec 12. J Virol. 2019. PMID: 31597765 Free PMC article.
-
Unique Infectious Strategy of H5N1 Avian Influenza Virus Is Governed by the Acid-Destabilized Property of Hemagglutinin.Viral Immunol. 2017 Jul/Aug;30(6):398-407. doi: 10.1089/vim.2017.0020. Epub 2017 Jun 27. Viral Immunol. 2017. PMID: 28654310 Review.
-
Hemagglutinin Stability and Its Impact on Influenza A Virus Infectivity, Pathogenicity, and Transmissibility in Avians, Mice, Swine, Seals, Ferrets, and Humans.Viruses. 2021 Apr 24;13(5):746. doi: 10.3390/v13050746. Viruses. 2021. PMID: 33923198 Free PMC article. Review.
Cited by
-
Animal models for the risk assessment of viral pandemic potential.Lab Anim Res. 2020 Apr 22;36:11. doi: 10.1186/s42826-020-00040-6. eCollection 2020. Lab Anim Res. 2020. PMID: 32337177 Free PMC article. Review.
-
Avian influenza A viruses: from zoonosis to pandemic.Future Virol. 2014 May 1;9(5):513-524. doi: 10.2217/fvl.14.30. Future Virol. 2014. PMID: 25214882 Free PMC article.
-
Evolution of Influenza A Virus by Mutation and Re-Assortment.Int J Mol Sci. 2017 Aug 7;18(8):1650. doi: 10.3390/ijms18081650. Int J Mol Sci. 2017. PMID: 28783091 Free PMC article. Review.
-
Divalent cation-induced conformational changes of influenza virus hemagglutinin.Sci Rep. 2020 Sep 22;10(1):15457. doi: 10.1038/s41598-020-72368-x. Sci Rep. 2020. PMID: 32963316 Free PMC article.
-
Mutations to PB2 and NP proteins of an avian influenza virus combine to confer efficient growth in primary human respiratory cells.J Virol. 2014 Nov;88(22):13436-46. doi: 10.1128/JVI.01093-14. Epub 2014 Sep 10. J Virol. 2014. PMID: 25210184 Free PMC article.
References
-
- Jagger BW, Wise HM, Kash JC, Walters KA, Wills NM, Xiao YL, Dunfee RL, Schwartzman LM, Ozinsky A, Bell GL, Dalton RM, Lo A, Efstathiou S, Atkins JF, Firth AE, Taubenberger JK, Digard P. 2012. An overlapping protein-coding region in influenza A virus segment 3 modulates the host response. Science 337:199–204 - PMC - PubMed
-
- Nelson MI, Holmes EC. 2007. The evolution of epidemic influenza. Nat. Rev. Genet. 8:196–205 - PubMed
Publication types
MeSH terms
Substances
Grants and funding
LinkOut - more resources
Full Text Sources
Other Literature Sources
Medical
Research Materials
Miscellaneous