Transcription under torsion
- PMID: 23812716
- PMCID: PMC5657242
- DOI: 10.1126/science.1235441
Transcription under torsion
Abstract
In cells, RNA polymerase (RNAP) must transcribe supercoiled DNA, whose torsional state is constantly changing, but how RNAP deals with DNA supercoiling remains elusive. We report direct measurements of individual Escherichia coli RNAPs as they transcribed supercoiled DNA. We found that a resisting torque slowed RNAP and increased its pause frequency and duration. RNAP was able to generate 11 ± 4 piconewton-nanometers (mean ± standard deviation) of torque before stalling, an amount sufficient to melt DNA of arbitrary sequence and establish RNAP as a more potent torsional motor than previously known. A stalled RNAP was able to resume transcription upon torque relaxation, and transcribing RNAP was resilient to transient torque fluctuations. These results provide a quantitative framework for understanding how dynamic modification of DNA supercoiling regulates transcription.
Figures
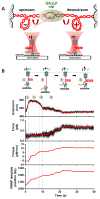
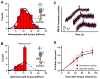
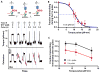
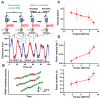
Comment in
-
RNA polymerase is a powerful torsional motor.Cell Cycle. 2014;13(3):337-8. doi: 10.4161/cc.27508. Epub 2013 Dec 13. Cell Cycle. 2014. PMID: 24335432 Free PMC article. No abstract available.
Similar articles
-
RNA polymerase is a powerful torsional motor.Cell Cycle. 2014;13(3):337-8. doi: 10.4161/cc.27508. Epub 2013 Dec 13. Cell Cycle. 2014. PMID: 24335432 Free PMC article. No abstract available.
-
On the stability of stalled RNA polymerase and its removal by RapA.Nucleic Acids Res. 2022 Jul 22;50(13):7396-7405. doi: 10.1093/nar/gkac558. Nucleic Acids Res. 2022. PMID: 35819188 Free PMC article.
-
A Mechanistic Model for Cooperative Behavior of Co-transcribing RNA Polymerases.PLoS Comput Biol. 2016 Aug 12;12(8):e1005069. doi: 10.1371/journal.pcbi.1005069. eCollection 2016 Aug. PLoS Comput Biol. 2016. PMID: 27517607 Free PMC article.
-
The Role of Supercoiling in the Motor Activity of RNA Polymerases.Methods Mol Biol. 2018;1805:215-232. doi: 10.1007/978-1-4939-8556-2_11. Methods Mol Biol. 2018. PMID: 29971720 Review.
-
Transcription factor dynamics.Microbiology (Reading). 2008 Jul;154(Pt 7):1837-1844. doi: 10.1099/mic.0.2008/018549-0. Microbiology (Reading). 2008. PMID: 18599813 Review.
Cited by
-
Nucleosomes undergo slow spontaneous gaping.Nucleic Acids Res. 2015 Apr 30;43(8):3964-71. doi: 10.1093/nar/gkv276. Epub 2015 Mar 30. Nucleic Acids Res. 2015. PMID: 25824950 Free PMC article.
-
Elucidating the Influence of Chromosomal Architecture on Transcriptional Regulation in Prokaryotes - Observing Strong Local Effects of Nucleoid Structure on Gene Regulation.Front Microbiol. 2020 Sep 1;11:2002. doi: 10.3389/fmicb.2020.02002. eCollection 2020. Front Microbiol. 2020. PMID: 32983020 Free PMC article.
-
RNA Polymerase II Regulates Topoisomerase 1 Activity to Favor Efficient Transcription.Cell. 2016 Apr 7;165(2):357-71. doi: 10.1016/j.cell.2016.02.036. Cell. 2016. PMID: 27058666 Free PMC article.
-
Tunable Elliptical Cylinders for Rotational Mechanical Studies of Single DNA Molecules.bioRxiv [Preprint]. 2024 Sep 27:2024.09.25.614944. doi: 10.1101/2024.09.25.614944. bioRxiv. 2024. Update in: Sci Adv. 2024 Dec 13;10(50):eadr4519. doi: 10.1126/sciadv.adr4519 PMID: 39386461 Free PMC article. Updated. Preprint.
-
Coupling Between Production of Ribosomal RNA and Maturation: Just at the Beginning.Front Mol Biosci. 2021 Oct 26;8:778778. doi: 10.3389/fmolb.2021.778778. eCollection 2021. Front Mol Biosci. 2021. PMID: 34765647 Free PMC article. Review.
References
-
- Travers A, Muskhelishvili G. Nat Rev Micro. 2005;3:157. - PubMed
-
- Kouzine F, Liu J, Sanford S, Chung HJ, Levens D. Nat Struct Mol Biol. 2004;11:1092. - PubMed
-
- Kouzine F, Sanford S, Elisha-Feil Z, Levens D. Nat Struct Mol Biol. 2008;15:146. - PubMed
-
- Matsumoto K, Hirose S. J Cell Sci. 2004;117:3797. - PubMed
Publication types
MeSH terms
Substances
Grants and funding
LinkOut - more resources
Full Text Sources
Other Literature Sources