Role of RNA interference (RNAi) in dengue virus replication and identification of NS4B as an RNAi suppressor
- PMID: 23741001
- PMCID: PMC3754049
- DOI: 10.1128/JVI.02774-12
Role of RNA interference (RNAi) in dengue virus replication and identification of NS4B as an RNAi suppressor
Abstract
RNA interference (RNAi) is an important antiviral defense response in plants and invertebrates; however, evidences for its contribution to mammalian antiviral defense are few. In the present study, we demonstrate the anti-dengue virus role of RNAi in mammalian cells. Dengue virus infection of Huh 7 cells decreased the mRNA levels of host RNAi factors, namely, Dicer, Drosha, Ago1, and Ago2, and in corollary, silencing of these genes in virus-infected cells enhanced dengue virus replication. In addition, we observed downregulation of many known human microRNAs (miRNAs) in response to viral infection. Using reversion-of-silencing assays, we further showed that NS4B of all four dengue virus serotypes is a potent RNAi suppressor. We generated a series of deletion mutants and demonstrated that NS4B mediates RNAi suppression via its middle and C-terminal domains, namely, transmembrane domain 3 (TMD3) and TMD5. Importantly, the NS4B N-terminal region, including the signal sequence 2K, which has been implicated in interferon (IFN)-antagonistic properties, was not involved in mediating RNAi suppressor activity. Site-directed mutagenesis of conserved residues revealed that a Phe-to-Ala (F112A) mutation in the TMD3 region resulted in a significant reduction of the RNAi suppression activity. The green fluorescent protein (GFP)-small interfering RNA (siRNA) biogenesis of the GFP-silenced line was considerably reduced by wild-type NS4B, while the F112A mutant abrogated this reduction. These results were further confirmed by in vitro dicer assays. Together, our results suggest the involvement of miRNA/RNAi pathways in dengue virus establishment and that dengue virus NS4B protein plays an important role in the modulation of the host RNAi/miRNA pathway to favor dengue virus replication.
Figures
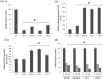
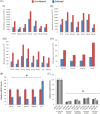
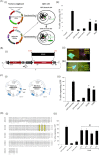
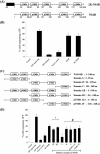
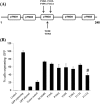
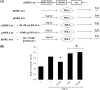
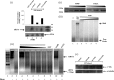
Similar articles
-
Mutation of Putative N-Glycosylation Sites on Dengue Virus NS4B Decreases RNA Replication.J Virol. 2015 Jul;89(13):6746-60. doi: 10.1128/JVI.00423-15. Epub 2015 Apr 15. J Virol. 2015. PMID: 25878113 Free PMC article.
-
A Combined Genetic-Proteomic Approach Identifies Residues within Dengue Virus NS4B Critical for Interaction with NS3 and Viral Replication.J Virol. 2015 Jul;89(14):7170-86. doi: 10.1128/JVI.00867-15. Epub 2015 Apr 29. J Virol. 2015. PMID: 25926641 Free PMC article.
-
A trade-off in replication in mosquito versus mammalian systems conferred by a point mutation in the NS4B protein of dengue virus type 4.Virology. 2003 Jul 20;312(1):222-32. doi: 10.1016/s0042-6822(03)00197-1. Virology. 2003. PMID: 12890635
-
The Dengue Virus Replication Complex: From RNA Replication to Protein-Protein Interactions to Evasion of Innate Immunity.Adv Exp Med Biol. 2018;1062:115-129. doi: 10.1007/978-981-10-8727-1_9. Adv Exp Med Biol. 2018. PMID: 29845529 Review.
-
Dengue virus NS4B protein as a target for developing antivirals.Front Cell Infect Microbiol. 2022 Aug 9;12:959727. doi: 10.3389/fcimb.2022.959727. eCollection 2022. Front Cell Infect Microbiol. 2022. PMID: 36017362 Free PMC article. Review.
Cited by
-
Rise and fall of vector infectivity during sequential strain displacements by mosquito-borne dengue virus.J Evol Biol. 2016 Nov;29(11):2205-2218. doi: 10.1111/jeb.12939. Epub 2016 Aug 8. J Evol Biol. 2016. PMID: 27500505 Free PMC article.
-
Innate Immune Antagonism of Mosquito-Borne Flaviviruses in Humans and Mosquitoes.Viruses. 2021 Oct 20;13(11):2116. doi: 10.3390/v13112116. Viruses. 2021. PMID: 34834923 Free PMC article. Review.
-
Mammalian antiviral systems directed by small RNA.PLoS Pathog. 2021 Dec 16;17(12):e1010091. doi: 10.1371/journal.ppat.1010091. eCollection 2021 Dec. PLoS Pathog. 2021. PMID: 34914813 Free PMC article. Review.
-
RNA Interference Restricts Rift Valley Fever Virus in Multiple Insect Systems.mSphere. 2017 May 3;2(3):e00090-17. doi: 10.1128/mSphere.00090-17. eCollection 2017 May-Jun. mSphere. 2017. PMID: 28497117 Free PMC article.
-
A Tale of Two RNAs during Viral Infection: How Viruses Antagonize mRNAs and Small Non-Coding RNAs in The Host Cell.Viruses. 2016 Jun 2;8(6):154. doi: 10.3390/v8060154. Viruses. 2016. PMID: 27271653 Free PMC article. Review.
References
-
- Ding SW. 2010. RNA-based antiviral immunity. Nat. Rev. Immunol. 10:632–644 - PubMed
Publication types
MeSH terms
Substances
LinkOut - more resources
Full Text Sources
Other Literature Sources