Iron homeostasis in the liver
- PMID: 23720289
- PMCID: PMC3936199
- DOI: 10.1002/cphy.c120016
Iron homeostasis in the liver
Abstract
Iron is an essential nutrient that is tightly regulated. A principal function of the liver is the regulation of iron homeostasis. The liver senses changes in systemic iron requirements and can regulate iron concentrations in a robust and rapid manner. The last 10 years have led to the discovery of several regulatory mechanisms in the liver that control the production of iron regulatory genes, storage capacity, and iron mobilization. Dysregulation of these functions leads to an imbalance of iron, which is the primary cause of iron-related disorders. Anemia and iron overload are two of the most prevalent disorders worldwide and affect over a billion people. Several mutations in liver-derived genes have been identified, demonstrating the central role of the liver in iron homeostasis. During conditions of excess iron, the liver increases iron storage and protects other tissues, namely, the heart and pancreas from iron-induced cellular damage. However, a chronic increase in liver iron stores results in excess reactive oxygen species production and liver injury. Excess liver iron is one of the major mechanisms leading to increased steatohepatitis, fibrosis, cirrhosis, and hepatocellular carcinoma.
Figures
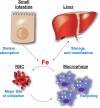
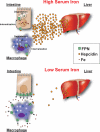
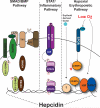
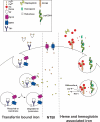
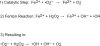
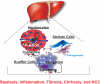
Similar articles
-
The Role of Iron and Iron Overload in Chronic Liver Disease.Med Sci Monit. 2016 Jun 22;22:2144-51. doi: 10.12659/msm.896494. Med Sci Monit. 2016. PMID: 27332079 Free PMC article. Review.
-
Molecular pathogenesis and clinical consequences of iron overload in liver cirrhosis.Hepatobiliary Pancreat Dis Int. 2016 Oct;15(5):461-479. doi: 10.1016/s1499-3872(16)60135-2. Hepatobiliary Pancreat Dis Int. 2016. PMID: 27733315 Review.
-
An ellagic acid isolated from Clerodendrum viscosum leaves ameliorates iron-overload induced hepatotoxicity in Swiss albino mice through inhibition of oxidative stress and the apoptotic pathway.Biomed Pharmacother. 2018 Oct;106:454-465. doi: 10.1016/j.biopha.2018.06.133. Epub 2018 Jul 11. Biomed Pharmacother. 2018. PMID: 29990833
-
Overview of iron metabolism in health and disease.Hemodial Int. 2017 Jun;21 Suppl 1(Suppl 1):S6-S20. doi: 10.1111/hdi.12542. Epub 2017 Mar 15. Hemodial Int. 2017. PMID: 28296010 Free PMC article. Review.
-
Mice lacking liver-specific β-catenin develop steatohepatitis and fibrosis after iron overload.J Hepatol. 2017 Aug;67(2):360-369. doi: 10.1016/j.jhep.2017.03.012. Epub 2017 Mar 22. J Hepatol. 2017. PMID: 28341391 Free PMC article.
Cited by
-
Melatonin Regulates Iron Homeostasis by Inducing Hepcidin Expression in Hepatocytes.Int J Mol Sci. 2022 Mar 25;23(7):3593. doi: 10.3390/ijms23073593. Int J Mol Sci. 2022. PMID: 35408955 Free PMC article.
-
Taurine Ameliorates Iron Overload-Induced Hepatocyte Injury via the Bcl-2/VDAC1-Mediated Mitochondrial Apoptosis Pathway.Oxid Med Cell Longev. 2022 Jul 16;2022:4135752. doi: 10.1155/2022/4135752. eCollection 2022. Oxid Med Cell Longev. 2022. Retraction in: Oxid Med Cell Longev. 2024 Jan 9;2024:9804902. doi: 10.1155/2024/9804902 PMID: 35879990 Free PMC article. Retracted.
-
Macrophages and the development and progression of non-alcoholic fatty liver disease.Front Immunol. 2023 Jun 12;14:1195699. doi: 10.3389/fimmu.2023.1195699. eCollection 2023. Front Immunol. 2023. PMID: 37377968 Free PMC article. Review.
-
Comparison of oral iron chelators in the management of transfusion-dependent β-thalassemia major based on serum ferritin and liver enzymes.F1000Res. 2023 Dec 19;12:154. doi: 10.12688/f1000research.128810.2. eCollection 2023. F1000Res. 2023. PMID: 39233713 Free PMC article.
-
Development of an iron overload HepG2 cell model using ferrous ammonium citrate.Sci Rep. 2023 Dec 8;13(1):21915. doi: 10.1038/s41598-023-49072-7. Sci Rep. 2023. PMID: 38081916 Free PMC article.
References
-
- Abboud S, Haile DJ. A novel mammalian iron-regulated protein involved in intracellular iron metabolism. J Biol Chem. 2000;275(26):19906–19912. - PubMed
-
- Aigner E, Theurl I, Theurl M, Lederer D, Haufe H, Dietze O, Strasser M, Datz C, Weiss G. Pathways underlying iron accumulation in human nonalcoholic fatty liver disease. The American journal of clinical nutrition. 2008;87(5):1374–1383. - PubMed
-
- Aisen P, Brown EB. Structure and function of transferrin. Progress in hematology. 1975:925–56. - PubMed
-
- Aisen P, Leibman A, Zweier J. Stoichiometric and site characteristics of the binding of iron to human transferrin. J Biol Chem. 1978;253(6):1930–1937. - PubMed
Publication types
MeSH terms
Substances
Grants and funding
LinkOut - more resources
Full Text Sources
Other Literature Sources
Medical