Electron counting and beam-induced motion correction enable near-atomic-resolution single-particle cryo-EM
- PMID: 23644547
- PMCID: PMC3684049
- DOI: 10.1038/nmeth.2472
Electron counting and beam-induced motion correction enable near-atomic-resolution single-particle cryo-EM
Abstract
In recent work with large high-symmetry viruses, single-particle electron cryomicroscopy (cryo-EM) has achieved the determination of near-atomic-resolution structures by allowing direct fitting of atomic models into experimental density maps. However, achieving this goal with smaller particles of lower symmetry remains challenging. Using a newly developed single electron-counting detector, we confirmed that electron beam-induced motion substantially degrades resolution, and we showed that the combination of rapid readout and nearly noiseless electron counting allow image blurring to be corrected to subpixel accuracy, restoring intrinsic image information to high resolution (Thon rings visible to ∼3 Å). Using this approach, we determined a 3.3-Å-resolution structure of an ∼700-kDa protein with D7 symmetry, the Thermoplasma acidophilum 20S proteasome, showing clear side-chain density. Our method greatly enhances image quality and data acquisition efficiency-key bottlenecks in applying near-atomic-resolution cryo-EM to a broad range of protein samples.
Conflict of interest statement
The authors declare competing financial interests: details are available in the online version of the paper.
Figures
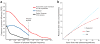
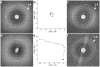
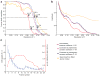
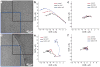
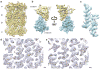
Comment in
-
Stroboscopic imaging of macromolecular complexes.Nat Methods. 2013 Jun;10(6):475-6. doi: 10.1038/nmeth.2486. Nat Methods. 2013. PMID: 23722205 No abstract available.
Similar articles
-
2.8 Å resolution reconstruction of the Thermoplasma acidophilum 20S proteasome using cryo-electron microscopy.Elife. 2015 Mar 11;4:e06380. doi: 10.7554/eLife.06380. Elife. 2015. PMID: 25760083 Free PMC article.
-
Cryo-EM single particle analysis with the Volta phase plate.Elife. 2016 Mar 7;5:e13046. doi: 10.7554/eLife.13046. Elife. 2016. PMID: 26949259 Free PMC article.
-
On the damage done to the structure of the Thermoplasma acidophilum proteasome by electron radiation.Protein Sci. 2018 Dec;27(12):2051-2061. doi: 10.1002/pro.3511. Epub 2018 Nov 5. Protein Sci. 2018. PMID: 30242932 Free PMC article.
-
Routine Collection of High-Resolution cryo-EM Datasets Using 200 KV Transmission Electron Microscope.J Vis Exp. 2022 Mar 16;(181). doi: 10.3791/63519. J Vis Exp. 2022. PMID: 35377368 Review.
-
Cryo-EM Structure Determination Using Segmented Helical Image Reconstruction.Methods Enzymol. 2016;579:307-28. doi: 10.1016/bs.mie.2016.05.034. Epub 2016 Jun 28. Methods Enzymol. 2016. PMID: 27572732 Review.
Cited by
-
Three-dimensional reconstruction of helical polymers.Arch Biochem Biophys. 2015 Sep 1;581:54-8. doi: 10.1016/j.abb.2015.04.004. Epub 2015 Apr 22. Arch Biochem Biophys. 2015. PMID: 25912526 Free PMC article. Review.
-
Visualization and Sequencing of Membrane Remodeling Leading to Influenza Virus Fusion.J Virol. 2016 Jul 11;90(15):6948-6962. doi: 10.1128/JVI.00240-16. Print 2016 Aug 1. J Virol. 2016. PMID: 27226364 Free PMC article.
-
CryoEM and mutagenesis reveal that the smallest capsid protein cements and stabilizes Kaposi's sarcoma-associated herpesvirus capsid.Proc Natl Acad Sci U S A. 2015 Feb 17;112(7):E649-56. doi: 10.1073/pnas.1420317112. Epub 2015 Feb 2. Proc Natl Acad Sci U S A. 2015. PMID: 25646489 Free PMC article.
-
Determination of the ribosome structure to a resolution of 2.5 Å by single-particle cryo-EM.Protein Sci. 2017 Jan;26(1):82-92. doi: 10.1002/pro.3068. Epub 2016 Oct 26. Protein Sci. 2017. PMID: 27750394 Free PMC article. Review.
-
Single-Molecule X-ray Scattering Used to Visualize the Conformation Distribution of Biological Molecules via Single-Object Scattering Sampling.Int J Mol Sci. 2023 Dec 5;24(24):17135. doi: 10.3390/ijms242417135. Int J Mol Sci. 2023. PMID: 38138965 Free PMC article.
References
Publication types
MeSH terms
Substances
Grants and funding
LinkOut - more resources
Full Text Sources
Other Literature Sources