Extracellular matrix aggregates from differentiating embryoid bodies as a scaffold to support ESC proliferation and differentiation
- PMID: 23637919
- PMCID: PMC3630218
- DOI: 10.1371/journal.pone.0061856
Extracellular matrix aggregates from differentiating embryoid bodies as a scaffold to support ESC proliferation and differentiation
Erratum in
- PLoS One. 2013;8(10). doi:10.1371/annotation/201f73d1-bf44-4528-b71c-b537aad520f3
Abstract
Embryonic stem cells (ESCs) have emerged as potential cell sources for tissue engineering and regeneration owing to its virtually unlimited replicative capacity and the potential to differentiate into a variety of cell types. Current differentiation strategies primarily involve various growth factor/inducer/repressor concoctions with less emphasis on the substrate. Developing biomaterials to promote stem cell proliferation and differentiation could aid in the realization of this goal. Extracellular matrix (ECM) components are important physiological regulators, and can provide cues to direct ESC expansion and differentiation. ECM undergoes constant remodeling with surrounding cells to accommodate specific developmental event. In this study, using ESC derived aggregates called embryoid bodies (EB) as a model, we characterized the biological nature of ECM in EB after exposure to different treatments: spontaneously differentiated and retinoic acid treated (denoted as SPT and RA, respectively). Next, we extracted this treatment-specific ECM by detergent decellularization methods (Triton X-100, DOC and SDS are compared). The resulting EB ECM scaffolds were seeded with undifferentiated ESCs using a novel cell seeding strategy, and the behavior of ESCs was studied. Our results showed that the optimized protocol efficiently removes cells while retaining crucial ECM and biochemical components. Decellularized ECM from SPT EB gave rise to a more favorable microenvironment for promoting ESC attachment, proliferation, and early differentiation, compared to native EB and decellularized ECM from RA EB. These findings suggest that various treatment conditions allow the formulation of unique ESC-ECM derived scaffolds to enhance ESC bioactivities, including proliferation and differentiation for tissue regeneration applications.
Conflict of interest statement
Figures
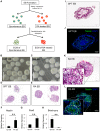
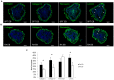
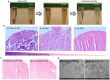
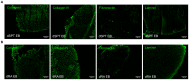
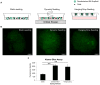
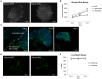
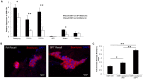
Similar articles
-
Crosslinking of extracellular matrix scaffolds derived from pluripotent stem cell aggregates modulates neural differentiation.Acta Biomater. 2016 Jan;30:222-232. doi: 10.1016/j.actbio.2015.11.016. Epub 2015 Nov 11. Acta Biomater. 2016. PMID: 26577988
-
Differential effects of acellular embryonic matrices on pluripotent stem cell expansion and neural differentiation.Biomaterials. 2015 Dec;73:231-42. doi: 10.1016/j.biomaterials.2015.09.020. Epub 2015 Sep 12. Biomaterials. 2015. PMID: 26410789
-
Recellularized rat testis scaffolds with embryoid bodies cells: a promising approach for tissue engineering.Syst Biol Reprod Med. 2022 Feb;68(1):44-54. doi: 10.1080/19396368.2021.2007554. Epub 2022 Jan 27. Syst Biol Reprod Med. 2022. PMID: 35086406
-
Engineering the embryoid body microenvironment to direct embryonic stem cell differentiation.Biotechnol Prog. 2009 Jan-Feb;25(1):43-51. doi: 10.1002/btpr.139. Biotechnol Prog. 2009. PMID: 19198003 Free PMC article. Review.
-
Decellularized extracellular matrix mediates tissue construction and regeneration.Front Med. 2022 Feb;16(1):56-82. doi: 10.1007/s11684-021-0900-3. Epub 2021 Dec 28. Front Med. 2022. PMID: 34962624 Free PMC article. Review.
Cited by
-
Decellularized Extracellular Matrix as an In Vitro Model to Study the Comprehensive Roles of the ECM in Stem Cell Differentiation.Stem Cells Int. 2016;2016:6397820. doi: 10.1155/2016/6397820. Epub 2015 Dec 6. Stem Cells Int. 2016. PMID: 26770210 Free PMC article. Review.
-
Towards Biomanufacturing of Cell-Derived Matrices.Int J Mol Sci. 2021 Nov 3;22(21):11929. doi: 10.3390/ijms222111929. Int J Mol Sci. 2021. PMID: 34769358 Free PMC article. Review.
-
Platr4 is an early embryonic lncRNA that exerts its function downstream on cardiogenic mesodermal lineage commitment.Dev Cell. 2022 Nov 7;57(21):2450-2468.e7. doi: 10.1016/j.devcel.2022.10.002. Dev Cell. 2022. PMID: 36347239 Free PMC article.
-
Bioengineering Thymus Organoids to Restore Thymic Function and Induce Donor-Specific Immune Tolerance to Allografts.Mol Ther. 2015 Jul;23(7):1262-1277. doi: 10.1038/mt.2015.77. Epub 2015 Apr 23. Mol Ther. 2015. PMID: 25903472 Free PMC article.
-
Loss of Ccbe1 affects cardiac-specification and cardiomyocyte differentiation in mouse embryonic stem cells.PLoS One. 2018 Oct 3;13(10):e0205108. doi: 10.1371/journal.pone.0205108. eCollection 2018. PLoS One. 2018. PMID: 30281646 Free PMC article.
References
-
- Doetschman TC, Eistetter H, Katz M, Schmidt W, Kemler R (1985) The in vitro development of blastocyst-derived embryonic stem cell lines: formation of visceral yolk sac, blood islands and myocardium. Journal of Embryology and Experimental Morphology 87: 27–&. - PubMed
-
- Daley WP, Peters SB, Larsen M (2008) Extracellular matrix dynamics in development and regenerative medicine. J Cell Sci 121: 255–264. - PubMed
Publication types
MeSH terms
Substances
Grants and funding
LinkOut - more resources
Full Text Sources
Other Literature Sources