Oligodendrocyte progenitors balance growth with self-repulsion to achieve homeostasis in the adult brain
- PMID: 23624515
- PMCID: PMC3807738
- DOI: 10.1038/nn.3390
Oligodendrocyte progenitors balance growth with self-repulsion to achieve homeostasis in the adult brain
Abstract
The adult CNS contains an abundant population of oligodendrocyte precursor cells (NG2(+) cells) that generate oligodendrocytes and repair myelin, but how these ubiquitous progenitors maintain their density is unknown. We generated NG2-mEGFP mice and used in vivo two-photon imaging to study their dynamics in the adult brain. Time-lapse imaging revealed that NG2(+) cells in the cortex were highly dynamic; they surveyed their local environment with motile filopodia, extended growth cones and continuously migrated. They maintained unique territories though self-avoidance, and NG2(+) cell loss though death, differentiation or ablation triggered rapid migration and proliferation of adjacent cells to restore their density. NG2(+) cells recruited to sites of focal CNS injury were similarly replaced by a proliferative burst surrounding the injury site. Thus, homeostatic control of NG2(+) cell density through a balance of active growth and self-repulsion ensures that these progenitors are available to replace oligodendrocytes and participate in tissue repair.
Figures
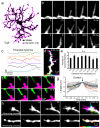
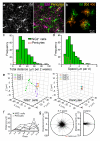
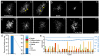
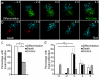
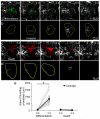
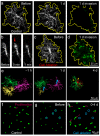
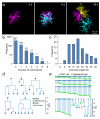
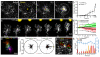
Similar articles
-
GPR17 expressing NG2-Glia: Oligodendrocyte progenitors serving as a reserve pool after injury.Glia. 2016 Feb;64(2):287-99. doi: 10.1002/glia.22929. Epub 2015 Oct 14. Glia. 2016. PMID: 26464068
-
NG2 glial cells regulate neuroimmunological responses to maintain neuronal function and survival.Sci Rep. 2017 Feb 14;7:42041. doi: 10.1038/srep42041. Sci Rep. 2017. PMID: 28195192 Free PMC article.
-
Progenitors in the adult cerebral cortex: cell cycle properties and regulation by physiological stimuli and injury.Glia. 2011 Jun;59(6):869-81. doi: 10.1002/glia.21156. Epub 2011 Mar 28. Glia. 2011. PMID: 21446038
-
Physiology of Oligodendroglia.Adv Exp Med Biol. 2019;1175:117-128. doi: 10.1007/978-981-13-9913-8_5. Adv Exp Med Biol. 2019. PMID: 31583586 Review.
-
NG2-glia, More Than Progenitor Cells.Adv Exp Med Biol. 2016;949:27-45. doi: 10.1007/978-3-319-40764-7_2. Adv Exp Med Biol. 2016. PMID: 27714683 Review.
Cited by
-
Neurotropic Murine β-Coronavirus Infection Causes Differential Expression of Connexin 47 in Oligodendrocyte Subpopulations Associated with Demyelination.Mol Neurobiol. 2024 Sep 18. doi: 10.1007/s12035-024-04482-0. Online ahead of print. Mol Neurobiol. 2024. PMID: 39292341
-
Radial glia inhibit peripheral glial infiltration into the spinal cord at motor exit point transition zones.Glia. 2016 Jul;64(7):1138-53. doi: 10.1002/glia.22987. Epub 2016 Mar 31. Glia. 2016. PMID: 27029762 Free PMC article.
-
The Secreted Glycoprotein Reelin Suppresses the Proliferation and Regulates the Distribution of Oligodendrocyte Progenitor Cells in the Embryonic Neocortex.J Neurosci. 2020 Sep 30;40(40):7625-7636. doi: 10.1523/JNEUROSCI.0125-20.2020. Epub 2020 Sep 10. J Neurosci. 2020. PMID: 32913108 Free PMC article.
-
Role of NG2 expressing cells in addiction: a new approach for an old problem.Front Pharmacol. 2014 Dec 19;5:279. doi: 10.3389/fphar.2014.00279. eCollection 2014. Front Pharmacol. 2014. PMID: 25566075 Free PMC article. Review.
-
Actin filament turnover drives leading edge growth during myelin sheath formation in the central nervous system.Dev Cell. 2015 Jul 27;34(2):139-151. doi: 10.1016/j.devcel.2015.05.013. Epub 2015 Jul 9. Dev Cell. 2015. PMID: 26166299 Free PMC article.
References
-
- Simons BD, Clevers H. Strategies for homeostatic stem cell self-renewal in adult tissues. Cell. 2011;145:851–862. - PubMed
-
- Zhao C, Deng W, Gage FH. Mechanisms and functional implications of adult neurogenesis. Cell. 2008;132:645–660. - PubMed
-
- Franklin RJ, Gilson JM, Blakemore WF. Local recruitment of remyelinating cells in the repair of demyelination in the central nervous system. J Neurosci Res. 1997;50:337–344. - PubMed
-
- Ajami B, Bennett JL, Krieger C, Tetzlaff W, Rossi FM. Local self-renewal can sustain CNS microglia maintenance and function throughout adult life. Nat Neurosci. 2007;10:1538–1543. - PubMed
Publication types
MeSH terms
Grants and funding
LinkOut - more resources
Full Text Sources
Other Literature Sources
Molecular Biology Databases
Research Materials