Tissue kallikrein-modified human endothelial progenitor cell implantation improves cardiac function via enhanced activation of akt and increased angiogenesis
- PMID: 23508045
- PMCID: PMC4051305
- DOI: 10.1038/labinvest.2013.48
Tissue kallikrein-modified human endothelial progenitor cell implantation improves cardiac function via enhanced activation of akt and increased angiogenesis
Abstract
Endothelial progenitor cells (EPCs) have been shown to enhance angiogenesis not only by incorporating into the vasculature but also by secreting cytokines, thereby serving as an ideal vehicle for gene transfer. As tissue kallikrein (TK) has pleiotropic effects in inhibiting apoptosis and oxidative stress, and promoting angiogenesis, we evaluated the salutary potential of kallikrein-modified human EPCs (hEPCs; Ad.hTK-hEPCs) after acute myocardial infarction (MI). We genetically modified hEPCs with a TK gene and evaluated cell survival, engraftment, revascularization, and functional improvement in a nude mouse left anterior descending ligation model. hEPCs were manipulated to overexpress the TK gene. In vitro, the antiapoptotic and paracrine effects were assessed under oxidative stress. TK protects hEPCs from oxidative stress-induced apoptosis via inhibition of activation of caspase-3 and -9, induction of Akt phosphorylation, and secretion of vascular endothelial growth factor. In vivo, the Ad.hTK-hEPCs were transplanted after MI via intracardiac injection. The surviving cells were tracked after transplantation using near-infrared optical imaging. Left ventricular (LV) function was evaluated by transthoracic echocardiography. Capillary density was quantified using immunohistochemical staining. Engrafted Ad.hTK-hEPCs exhibited advanced protection against ischemia by increasing LV ejection fraction. Compared with Ad.Null-hEPCs, transplantation with Ad.hTK-hEPCs significantly decreased cardiomyocyte apoptosis in association with increased retention of transplanted EPCs in the myocardium. Capillary density and arteriolar density in the infarct border zone was significantly higher in Ad.hTK-hEPC-transplanted mice than in Ad.Null-hEPC-treated mice. Transplanted hEPCs were clearly incorporated into CD31(+) capillaries. These results indicate that implantation of kallikrein-modified EPCs in the heart provides advanced benefits in protection against ischemia-induced MI by enhanced angiogenesis and reducing apoptosis.
Conflict of interest statement
Figures
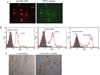
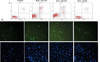
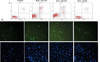
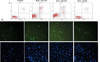
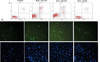
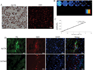
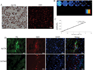
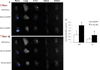
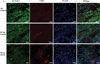
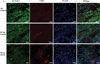
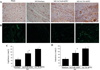
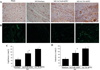
Similar articles
-
Tissue kallikrein promotes cardiac neovascularization by enhancing endothelial progenitor cell functional capacity.Hum Gene Ther. 2012 Aug;23(8):859-70. doi: 10.1089/hum.2011.123. Epub 2012 Apr 30. Hum Gene Ther. 2012. PMID: 22435954 Free PMC article.
-
Bradykinin preconditioning improves therapeutic potential of human endothelial progenitor cells in infarcted myocardium.PLoS One. 2013 Dec 2;8(12):e81505. doi: 10.1371/journal.pone.0081505. eCollection 2013. PLoS One. 2013. PMID: 24312554 Free PMC article.
-
Tissue kallikrein promotes neovascularization and improves cardiac function by the Akt-glycogen synthase kinase-3beta pathway.Cardiovasc Res. 2008 Dec 1;80(3):354-64. doi: 10.1093/cvr/cvn223. Epub 2008 Aug 9. Cardiovasc Res. 2008. PMID: 18689794 Free PMC article.
-
Regional and global protective effects of tissue kallikrein gene delivery to the peri-infarct myocardium.Regen Med. 2006 Mar;1(2):235-54. doi: 10.2217/17460751.1.2.235. Regen Med. 2006. PMID: 17465807
-
Akt/protein kinase B and endothelial nitric oxide synthase mediate muscular neovascularization induced by tissue kallikrein gene transfer.Circulation. 2004 Sep 21;110(12):1638-44. doi: 10.1161/01.CIR.0000142051.36244.83. Epub 2004 Sep 13. Circulation. 2004. PMID: 15364809
Cited by
-
Novel role of kallistatin in vascular repair by promoting mobility, viability, and function of endothelial progenitor cells.J Am Heart Assoc. 2014 Sep 18;3(5):e001194. doi: 10.1161/JAHA.114.001194. J Am Heart Assoc. 2014. PMID: 25237049 Free PMC article.
-
Bradykinin inhibits oxidative stress-induced senescence of endothelial progenitor cells through the B2R/AKT/RB and B2R/EGFR/RB signal pathways.Oncotarget. 2015 Sep 22;6(28):24675-89. doi: 10.18632/oncotarget.5071. Oncotarget. 2015. PMID: 26360782 Free PMC article.
-
Enhancing endothelial progenitor cell for clinical use.World J Stem Cells. 2015 Jul 26;7(6):894-8. doi: 10.4252/wjsc.v7.i6.894. World J Stem Cells. 2015. PMID: 26240678 Free PMC article.
-
Genetically‑modified stem cells in treatment of human diseases: Tissue kallikrein (KLK1)‑based targeted therapy (Review).Int J Mol Med. 2018 Mar;41(3):1177-1186. doi: 10.3892/ijmm.2018.3361. Epub 2018 Jan 3. Int J Mol Med. 2018. PMID: 29328364 Free PMC article. Review.
-
Pharmacological effects of recombinant human tissue kallikrein on bradykinin B2 receptors.Pharmacol Res Perspect. 2015 Mar;3(2):e00119. doi: 10.1002/prp2.119. Epub 2015 Feb 10. Pharmacol Res Perspect. 2015. PMID: 26038695 Free PMC article.
References
-
- Schuh A, Liehn EA, Sasse A, et al. Transplantation of endothelial progenitor cells improves neovascularization and left ventricular function after myocardial infarction in a rat model. Basic Res Cardiol. 2008;103:69–77. - PubMed
-
- Leone AM, Valgimigli M, Giannico MB, et al. From bone marrow to the arterial wall: the ongoing tale of endothelial progenitor cells. Eur Heart J. 2009;30:890–899. - PubMed
-
- Mangi AA, Noiseux N, Kong D, et al. Mesenchymal stem cells modified with Akt prevent remodeling and restore performance of infarcted hearts. Nat Med. 2003;9:1195–1201. - PubMed
Publication types
MeSH terms
Substances
Grants and funding
LinkOut - more resources
Full Text Sources
Other Literature Sources
Medical
Research Materials
Miscellaneous