Mapping the subcellular distribution of α-synuclein in neurons using genetically encoded probes for correlated light and electron microscopy: implications for Parkinson's disease pathogenesis
- PMID: 23392688
- PMCID: PMC3711410
- DOI: 10.1523/JNEUROSCI.2898-12.2013
Mapping the subcellular distribution of α-synuclein in neurons using genetically encoded probes for correlated light and electron microscopy: implications for Parkinson's disease pathogenesis
Abstract
Modifications to the gene encoding human α-synuclein have been linked to the development of Parkinson's disease. The highly conserved structure of α-synuclein suggests a functional interaction with membranes, and several lines of evidence point to a role in vesicle-related processes within nerve terminals. Using recombinant fusions of human α-synuclein, including new genetic tags developed for correlated light microscopy and electron microscopy (the tetracysteine-biarsenical labeling system or the new fluorescent protein for electron microscopy, MiniSOG), we determined the distribution of α-synuclein when overexpressed in primary neurons at supramolecular and cellular scales in three dimensions (3D). We observed specific association of α-synuclein with a large and otherwise poorly characterized membranous organelle system of the presynaptic terminal, as well as with smaller vesicular structures within these boutons. Furthermore, α-synuclein was localized to multiple elements of the protein degradation pathway, including multivesicular bodies in the axons and lysosomes within neuronal cell bodies. Examination of synapses in brains of transgenic mice overexpressing human α-synuclein revealed alterations of the presynaptic endomembrane systems similar to our findings in cell culture. Three-dimensional electron tomographic analysis of enlarged presynaptic terminals in several brain areas revealed that these terminals were filled with membrane-bounded organelles, including tubulovesicular structures similar to what we observed in vitro. We propose that α-synuclein overexpression is associated with hypertrophy of membrane systems of the presynaptic terminal previously shown to have a role in vesicle recycling. Our data support the conclusion that α-synuclein is involved in processes associated with the sorting, channeling, packaging, and transport of synaptic material destined for degradation.
Figures
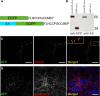
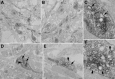
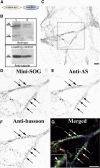
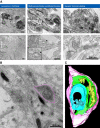
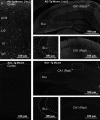
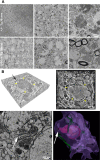
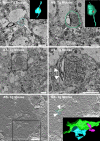
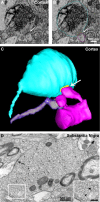
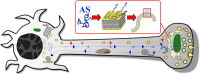
Similar articles
-
Presynaptic alpha-synuclein aggregation in a mouse model of Parkinson's disease.J Neurosci. 2014 Feb 5;34(6):2037-50. doi: 10.1523/JNEUROSCI.2581-13.2014. J Neurosci. 2014. PMID: 24501346 Free PMC article.
-
Direct evidence for ultrastructures of the α-synuclein-associated synaptic vesicle pool in presynaptic terminals.Biochim Biophys Acta Mol Basis Dis. 2024 Dec;1870(8):167494. doi: 10.1016/j.bbadis.2024.167494. Epub 2024 Sep 2. Biochim Biophys Acta Mol Basis Dis. 2024. PMID: 39233262
-
Alpha-synuclein-immunopositive myenteric neurons and vagal preganglionic terminals: autonomic pathway implicated in Parkinson's disease?Neuroscience. 2008 May 15;153(3):733-50. doi: 10.1016/j.neuroscience.2008.02.074. Epub 2008 Mar 18. Neuroscience. 2008. PMID: 18407422 Free PMC article.
-
Is Cell Death Primary or Secondary in the Pathophysiology of Idiopathic Parkinson's Disease?Biomolecules. 2015 Jul 16;5(3):1467-79. doi: 10.3390/biom5031467. Biomolecules. 2015. PMID: 26193328 Free PMC article. Review.
-
The Synaptic Function of α-Synuclein.J Parkinsons Dis. 2015;5(4):699-713. doi: 10.3233/JPD-150642. J Parkinsons Dis. 2015. PMID: 26407041 Free PMC article. Review.
Cited by
-
G2019S-LRRK2 Expression Augments α-Synuclein Sequestration into Inclusions in Neurons.J Neurosci. 2016 Jul 13;36(28):7415-27. doi: 10.1523/JNEUROSCI.3642-15.2016. J Neurosci. 2016. PMID: 27413152 Free PMC article.
-
Unconventional tonicity-regulated nuclear trafficking of NFAT5 mediated by KPNB1, XPOT and RUVBL2.J Cell Sci. 2022 Jul 1;135(13):jcs259280. doi: 10.1242/jcs.259280. Epub 2022 Jul 12. J Cell Sci. 2022. PMID: 35635291 Free PMC article.
-
Genetics and genomics of Parkinson's disease.Genome Med. 2014 Jun 30;6(6):48. doi: 10.1186/gm566. eCollection 2014. Genome Med. 2014. PMID: 25061481 Free PMC article. Review.
-
Genetically targeted 3D visualisation of Drosophila neurons under Electron Microscopy and X-Ray Microscopy using miniSOG.Sci Rep. 2016 Dec 13;6:38863. doi: 10.1038/srep38863. Sci Rep. 2016. PMID: 27958322 Free PMC article.
-
Effects of Excess Brain-Derived Human α-Synuclein on Synaptic Vesicle Trafficking.Front Neurosci. 2021 Feb 4;15:639414. doi: 10.3389/fnins.2021.639414. eCollection 2021. Front Neurosci. 2021. PMID: 33613189 Free PMC article.
References
-
- Braak H, Del Tredici K, Rüb U, de Vos RA, Jansen Steur EN, Braak E. Staging of brain pathology related to sporadic Parkinson's disease. Neurobiol Aging. 2003;24:197–211. - PubMed
-
- Chartier-Harlin MC, Kachergus J, Roumier C, Mouroux V, Douay X, Lincoln S, Levecque C, Larvor L, Andrieux J, Hulihan M, Waucquier N, Defebvre L, Amouyel P, Farrer M, Destée A. Alpha-synuclein locus duplication as a cause of familial Parkinson's disease. Lancet. 2004;364:1167–1169. - PubMed
-
- Danzer KM, Krebs SK, Wolff M, Birk G, Hengerer B. Seeding induced by alpha-synuclein oligomers provides evidence for spreading of alpha-synuclein pathology. J Neurochem. 2009;111:192–203. - PubMed
Publication types
MeSH terms
Substances
Grants and funding
- P41 GM103412/GM/NIGMS NIH HHS/United States
- 5P41RR004050-24/RR/NCRR NIH HHS/United States
- R25 DA026401/DA/NIDA NIH HHS/United States
- R01 GM086197-05/GM/NIGMS NIH HHS/United States
- P41 RR004050/RR/NCRR NIH HHS/United States
- AG022074/AG/NIA NIH HHS/United States
- P01 AG022074/AG/NIA NIH HHS/United States
- 8 P41 GM103412-24/GM/NIGMS NIH HHS/United States
- R01 GM086197/GM/NIGMS NIH HHS/United States
- AG184440/AG/NIA NIH HHS/United States
- R37 AG018440/AG/NIA NIH HHS/United States
- R01 NS027177/NS/NINDS NIH HHS/United States
- P01 AG010435/AG/NIA NIH HHS/United States
LinkOut - more resources
Full Text Sources
Other Literature Sources
Medical
Molecular Biology Databases
Miscellaneous