RIAM and vinculin binding to talin are mutually exclusive and regulate adhesion assembly and turnover
- PMID: 23389036
- PMCID: PMC3605642
- DOI: 10.1074/jbc.M112.438119
RIAM and vinculin binding to talin are mutually exclusive and regulate adhesion assembly and turnover
Abstract
Talin activates integrins, couples them to F-actin, and recruits vinculin to focal adhesions (FAs). Here, we report the structural characterization of the talin rod: 13 helical bundles (R1-R13) organized into a compact cluster of four-helix bundles (R2-R4) within a linear chain of five-helix bundles. Nine of the bundles contain vinculin-binding sites (VBS); R2R3 are atypical, with each containing two VBS. Talin R2R3 also binds synergistically to RIAM, a Rap1 effector involved in integrin activation. Biochemical and structural data show that vinculin and RIAM binding to R2R3 is mutually exclusive. Moreover, vinculin binding requires domain unfolding, whereas RIAM binds the folded R2R3 double domain. In cells, RIAM is enriched in nascent adhesions at the leading edge whereas vinculin is enriched in FAs. We propose a model in which RIAM binding to R2R3 initially recruits talin to membranes where it activates integrins. As talin engages F-actin, force exerted on R2R3 disrupts RIAM binding and exposes the VBS, which recruit vinculin to stabilize the complex.
Figures
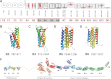
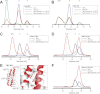
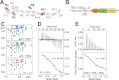
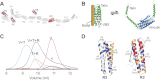
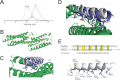
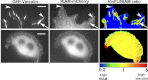
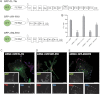
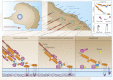
Similar articles
-
Pressure-Dependent Chemical Shifts in the R3 Domain of Talin Show that It Is Thermodynamically Poised for Binding to Either Vinculin or RIAM.Structure. 2017 Dec 5;25(12):1856-1866.e2. doi: 10.1016/j.str.2017.10.008. Epub 2017 Nov 16. Structure. 2017. PMID: 29153504
-
Two modes of integrin activation form a binary molecular switch in adhesion maturation.Mol Biol Cell. 2013 May;24(9):1354-62. doi: 10.1091/mbc.E12-09-0695. Epub 2013 Mar 6. Mol Biol Cell. 2013. PMID: 23468527 Free PMC article.
-
Structural basis for amplifying vinculin activation by talin.J Biol Chem. 2004 Jun 25;279(26):27667-78. doi: 10.1074/jbc.M403076200. Epub 2004 Apr 7. J Biol Chem. 2004. PMID: 15070891
-
Integrin connections to the cytoskeleton through talin and vinculin.Biochem Soc Trans. 2008 Apr;36(Pt 2):235-9. doi: 10.1042/BST0360235. Biochem Soc Trans. 2008. PMID: 18363566 Review.
-
The Rap1-RIAM-talin axis of integrin activation and blood cell function.Blood. 2016 Jul 28;128(4):479-87. doi: 10.1182/blood-2015-12-638700. Epub 2016 May 20. Blood. 2016. PMID: 27207789 Free PMC article. Review.
Cited by
-
High-resolution snapshots of the talin auto-inhibitory states suggest roles in cell adhesion and signaling.Nat Commun. 2024 Oct 28;15(1):9270. doi: 10.1038/s41467-024-52581-2. Nat Commun. 2024. PMID: 39468080 Free PMC article.
-
Pre-complexation of talin and vinculin without tension is required for efficient nascent adhesion maturation.Elife. 2021 Mar 30;10:e66151. doi: 10.7554/eLife.66151. Elife. 2021. PMID: 33783351 Free PMC article.
-
Phosphatidylinositol 4,5-Bisphosphate Modulates the Affinity of Talin-1 for Phospholipid Bilayers and Activates Its Autoinhibited Form.Biochemistry. 2016 Sep 13;55(36):5038-48. doi: 10.1021/acs.biochem.6b00497. Epub 2016 Aug 31. Biochemistry. 2016. PMID: 27548281 Free PMC article.
-
Conformational equilibrium of talin is regulated by anionic lipids.Biochim Biophys Acta. 2016 Aug;1858(8):1833-40. doi: 10.1016/j.bbamem.2016.05.005. Epub 2016 May 6. Biochim Biophys Acta. 2016. PMID: 27163494 Free PMC article.
-
Different Vinculin Binding Sites Use the Same Mechanism to Regulate Directional Force Transduction.Biophys J. 2020 Mar 24;118(6):1344-1356. doi: 10.1016/j.bpj.2019.12.042. Epub 2020 Feb 4. Biophys J. 2020. PMID: 32109366 Free PMC article.
References
Publication types
MeSH terms
Substances
Associated data
- Actions
- Actions
- Actions
- Actions
- Actions
Grants and funding
LinkOut - more resources
Full Text Sources
Other Literature Sources
Molecular Biology Databases
Research Materials
Miscellaneous