Strigolactone can promote or inhibit shoot branching by triggering rapid depletion of the auxin efflux protein PIN1 from the plasma membrane
- PMID: 23382651
- PMCID: PMC3558495
- DOI: 10.1371/journal.pbio.1001474
Strigolactone can promote or inhibit shoot branching by triggering rapid depletion of the auxin efflux protein PIN1 from the plasma membrane
Abstract
Plants continuously extend their root and shoot systems through the action of meristems at their growing tips. By regulating which meristems are active, plants adjust their body plans to suit local environmental conditions. The transport network of the phytohormone auxin has been proposed to mediate this systemic growth coordination, due to its self-organising, environmentally sensitive properties. In particular, a positive feedback mechanism termed auxin transport canalization, which establishes auxin flow from active shoot meristems (auxin sources) to the roots (auxin sinks), has been proposed to mediate competition between shoot meristems and to balance shoot and root growth. Here we provide strong support for this hypothesis by demonstrating that a second hormone, strigolactone, regulates growth redistribution in the shoot by rapidly modulating auxin transport. A computational model in which strigolactone action is represented as an increase in the rate of removal of the auxin export protein, PIN1, from the plasma membrane can reproduce both the auxin transport and shoot branching phenotypes observed in various mutant combinations and strigolactone treatments, including the counterintuitive ability of strigolactones either to promote or inhibit shoot branching, depending on the auxin transport status of the plant. Consistent with this predicted mode of action, strigolactone signalling was found to trigger PIN1 depletion from the plasma membrane of xylem parenchyma cells in the stem. This effect could be detected within 10 minutes of strigolactone treatment and was independent of protein synthesis but dependent on clathrin-mediated membrane trafficking. Together these results support the hypothesis that growth across the plant shoot system is balanced by competition between shoot apices for a common auxin transport path to the root and that strigolactones regulate shoot branching by modulating this competition.
Conflict of interest statement
The authors have declared that no competing interests exist.
Figures
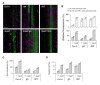
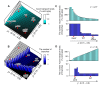
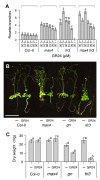
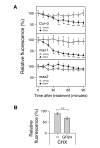
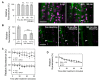
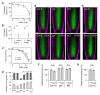
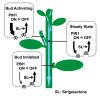
Comment in
-
Transforming a stem into a bush.PLoS Biol. 2013;11(1):e1001476. doi: 10.1371/journal.pbio.1001476. Epub 2013 Jan 29. PLoS Biol. 2013. PMID: 23382653 Free PMC article. No abstract available.
Similar articles
-
Connective auxin transport contributes to strigolactone-mediated shoot branching control independent of the transcription factor BRC1.PLoS Genet. 2019 Mar 13;15(3):e1008023. doi: 10.1371/journal.pgen.1008023. eCollection 2019 Mar. PLoS Genet. 2019. PMID: 30865619 Free PMC article.
-
The Arabidopsis MAX pathway controls shoot branching by regulating auxin transport.Curr Biol. 2006 Mar 21;16(6):553-63. doi: 10.1016/j.cub.2006.01.058. Curr Biol. 2006. PMID: 16546078
-
Strigolactones enhance competition between shoot branches by dampening auxin transport.Development. 2010 Sep 1;137(17):2905-13. doi: 10.1242/dev.051987. Epub 2010 Jul 28. Development. 2010. PMID: 20667910
-
The importance of strigolactone transport regulation for symbiotic signaling and shoot branching.Planta. 2016 Jun;243(6):1351-60. doi: 10.1007/s00425-016-2503-9. Epub 2016 Apr 4. Planta. 2016. PMID: 27040840 Free PMC article. Review.
-
Cellular events of strigolactone signalling and their crosstalk with auxin in roots.J Exp Bot. 2015 Aug;66(16):4855-61. doi: 10.1093/jxb/erv178. Epub 2015 Apr 21. J Exp Bot. 2015. PMID: 25900617 Review.
Cited by
-
Modulating root system architecture: cross-talk between auxin and phytohormones.Front Plant Sci. 2024 Feb 8;15:1343928. doi: 10.3389/fpls.2024.1343928. eCollection 2024. Front Plant Sci. 2024. PMID: 38390293 Free PMC article. Review.
-
Three ancient hormonal cues co-ordinate shoot branching in a moss.Elife. 2015 Mar 25;4:e06808. doi: 10.7554/eLife.06808. Elife. 2015. PMID: 25806686 Free PMC article.
-
Photosynthate Regulation of the Root System Architecture Mediated by the Heterotrimeric G Protein Complex in Arabidopsis.Front Plant Sci. 2016 Aug 25;7:1255. doi: 10.3389/fpls.2016.01255. eCollection 2016. Front Plant Sci. 2016. PMID: 27610112 Free PMC article.
-
BIG Modulates Stem Cell Niche and Meristem Development via SCR/SHR Pathway in Arabidopsis Roots.Int J Mol Sci. 2022 Jun 17;23(12):6784. doi: 10.3390/ijms23126784. Int J Mol Sci. 2022. PMID: 35743225 Free PMC article.
-
Masks Start to Drop: Suppressor of MAX2 1-Like Proteins Reveal Their Many Faces.Front Plant Sci. 2022 May 12;13:887232. doi: 10.3389/fpls.2022.887232. eCollection 2022. Front Plant Sci. 2022. PMID: 35645992 Free PMC article. Review.
References
-
- Leyser O (2009) The control of shoot branching: an example of plant information processing. Plant Cell Environ 32: 694–703. - PubMed
-
- Petrášek J, Mravec J, Bouchard R, Blakeslee JJ, Abas M, et al. (2006) PIN proteins perform a rate-limiting function in cellular auxin efflux. Science 312: 914–918. - PubMed
-
- Wiśniewska J, Xu J, Seifertová D, Brewer PB, Růžička K, et al. (2006) Polar PIN localization directs auxin flow in plants. Science 312: 883. - PubMed
-
- Gälweiler L, Guan C, Müller A, Wisman E, Mendgen K, et al. (1998) Regulation of polar auxin transport by AtPIN1 in Arabidopsis vascular tissue. Science 282: 2226–2230. - PubMed
Publication types
MeSH terms
Substances
Grants and funding
LinkOut - more resources
Full Text Sources
Other Literature Sources
Molecular Biology Databases
Miscellaneous