Factors shaping the adaptive landscape for arboviruses: implications for the emergence of disease
- PMID: 23374123
- PMCID: PMC3621119
- DOI: 10.2217/fmb.12.139
Factors shaping the adaptive landscape for arboviruses: implications for the emergence of disease
Abstract
Many examples of the emergence or re-emergence of infectious diseases involve the adaptation of zoonotic viruses to new amplification hosts or to humans themselves. These include several instances of simple mutational adaptations, often to hosts closely related to the natural reservoirs. However, based on theoretical grounds, arthropod-borne viruses, or arboviruses, may face several challenges for adaptation to new hosts. Here, we review recent findings regarding adaptive evolution of arboviruses and its impact on disease emergence. We focus on the zoonotic alphaviruses Venezuelan equine encephalitis and chikungunya viruses, which have undergone adaptive evolution that mediated recent outbreaks of disease, as well as the flaviviruses dengue and West Nile viruses, which have emerged via less dramatic adaptive mechanisms.
Figures
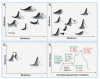
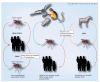
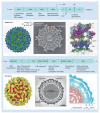
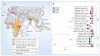
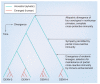
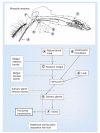
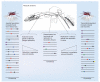
Similar articles
-
Transmission cycles, host range, evolution and emergence of arboviral disease.Nat Rev Microbiol. 2004 Oct;2(10):789-801. doi: 10.1038/nrmicro1006. Nat Rev Microbiol. 2004. PMID: 15378043 Free PMC article. Review.
-
Arboviruses pathogenic for domestic and wild animals.Adv Virus Res. 2014;89:201-75. doi: 10.1016/B978-0-12-800172-1.00005-7. Adv Virus Res. 2014. PMID: 24751197
-
Arboviral bottlenecks and challenges to maintaining diversity and fitness during mosquito transmission.Viruses. 2014 Oct 23;6(10):3991-4004. doi: 10.3390/v6103991. Viruses. 2014. PMID: 25341663 Free PMC article. Review.
-
The Chikungunya threat: an ecological and evolutionary perspective.Trends Microbiol. 2008 Feb;16(2):80-8. doi: 10.1016/j.tim.2007.12.003. Epub 2008 Jan 10. Trends Microbiol. 2008. PMID: 18191569 Review.
-
Human Urban Arboviruses Can Infect Wild Animals and Jump to Sylvatic Maintenance Cycles in South America.Front Cell Infect Microbiol. 2019 Jul 17;9:259. doi: 10.3389/fcimb.2019.00259. eCollection 2019. Front Cell Infect Microbiol. 2019. PMID: 31380302 Free PMC article. Review.
Cited by
-
Antiviral Activity of a Novel Compound CW-33 against Japanese Encephalitis Virus through Inhibiting Intracellular Calcium Overload.Int J Mol Sci. 2016 Aug 24;17(9):1386. doi: 10.3390/ijms17091386. Int J Mol Sci. 2016. PMID: 27563890 Free PMC article.
-
Aedes-borne orthoflavivirus infections in neotropical primates - Ecology, susceptibility, and pathogenesis.Exp Biol Med (Maywood). 2023 Nov;248(22):2030-2038. doi: 10.1177/15353702231220659. Epub 2024 Jan 17. Exp Biol Med (Maywood). 2023. PMID: 38230520 Free PMC article. Review.
-
Intra-Host Diversity of Dengue Virus in Mosquito Vectors.Front Cell Infect Microbiol. 2022 Jun 22;12:888804. doi: 10.3389/fcimb.2022.888804. eCollection 2022. Front Cell Infect Microbiol. 2022. PMID: 35811685 Free PMC article. Review.
-
Alphavirus mutator variants present host-specific defects and attenuation in mammalian and insect models.PLoS Pathog. 2014 Jan;10(1):e1003877. doi: 10.1371/journal.ppat.1003877. Epub 2014 Jan 16. PLoS Pathog. 2014. PMID: 24453971 Free PMC article.
-
Population genetic structure of Culex tritaeniorhynchus in different types of climatic zones in China.BMC Genomics. 2024 Jul 5;25(1):673. doi: 10.1186/s12864-024-10589-4. BMC Genomics. 2024. PMID: 38969975 Free PMC article.
References
-
- Balboni A, Battilani M, Prosperi S. The SARS-like coronaviruses. the role of bats and evolutionary relationships with SARS coronavirus. New Microbiol. 2012;35(1):1–16. - PubMed
Publication types
MeSH terms
Grants and funding
LinkOut - more resources
Full Text Sources
Other Literature Sources
Medical