GATA3 suppresses metastasis and modulates the tumour microenvironment by regulating microRNA-29b expression
- PMID: 23354167
- PMCID: PMC3660859
- DOI: 10.1038/ncb2672
GATA3 suppresses metastasis and modulates the tumour microenvironment by regulating microRNA-29b expression
Abstract
Despite advances in our understanding of breast cancer, patients with metastatic disease have poor prognoses. GATA3 is a transcription factor that specifies and maintains mammary luminal epithelial cell fate, and its expression is lost in breast cancer, correlating with a worse prognosis in human patients. Here, we show that GATA3 promotes differentiation, suppresses metastasis and alters the tumour microenvironment in breast cancer by inducing microRNA-29b (miR-29b) expression. Accordingly, miR-29b is enriched in luminal breast cancers and loss of miR-29b, even in GATA3-expressing cells, increases metastasis and promotes a mesenchymal phenotype. Mechanistically, miR-29b inhibits metastasis by targeting a network of pro-metastatic regulators involved in angiogenesis, collagen remodelling and proteolysis, including VEGFA, ANGPTL4, PDGF, LOX and MMP9, and targeting ITGA6, ITGB1 and TGFB, thereby indirectly affecting differentiation and epithelial plasticity. The discovery that a GATA3-miR-29b axis regulates the tumour microenvironment and inhibits metastasis opens up possibilities for therapeutic intervention in breast cancer.
Figures
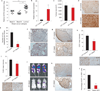
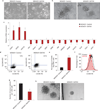
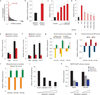
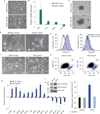
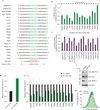
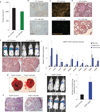
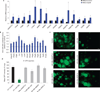
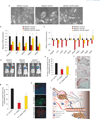
Comment in
-
miR-29b moulds the tumour microenvironment to repress metastasis.Nat Cell Biol. 2013 Feb;15(2):139-40. doi: 10.1038/ncb2684. Nat Cell Biol. 2013. PMID: 23377028
Similar articles
-
miR-29b moulds the tumour microenvironment to repress metastasis.Nat Cell Biol. 2013 Feb;15(2):139-40. doi: 10.1038/ncb2684. Nat Cell Biol. 2013. PMID: 23377028
-
MicroRNA-455-3p mediates GATA3 tumor suppression in mammary epithelial cells by inhibiting TGF-β signaling.J Biol Chem. 2019 Oct 25;294(43):15808-15825. doi: 10.1074/jbc.RA119.010800. Epub 2019 Sep 6. J Biol Chem. 2019. PMID: 31492753 Free PMC article.
-
GATA3 inhibits breast cancer growth and pulmonary breast cancer metastasis.Oncogene. 2009 Jul 23;28(29):2634-42. doi: 10.1038/onc.2009.126. Epub 2009 Jun 1. Oncogene. 2009. PMID: 19483726
-
GATA3 in development and cancer differentiation: cells GATA have it!J Cell Physiol. 2010 Jan;222(1):42-9. doi: 10.1002/jcp.21943. J Cell Physiol. 2010. PMID: 19798694 Free PMC article. Review.
-
Gene of the month: GATA3.J Clin Pathol. 2023 Dec;76(12):793-797. doi: 10.1136/jcp-2023-209017. Epub 2023 Sep 19. J Clin Pathol. 2023. PMID: 37726118 Review.
Cited by
-
Cyclin E overexpression confers resistance to trastuzumab through noncanonical phosphorylation of SMAD3 in HER2+ breast cancer.Cancer Biol Ther. 2020 Nov 1;21(11):994-1004. doi: 10.1080/15384047.2020.1818518. Epub 2020 Oct 14. Cancer Biol Ther. 2020. PMID: 33054513 Free PMC article.
-
MicroRNA-16 suppresses metastasis in an orthotopic, but not autochthonous, mouse model of soft tissue sarcoma.Dis Model Mech. 2015 Aug 1;8(8):867-75. doi: 10.1242/dmm.017897. Epub 2015 Jun 4. Dis Model Mech. 2015. PMID: 26044957 Free PMC article.
-
Involvement of DNMT 3B promotes epithelial-mesenchymal transition and gene expression profile of invasive head and neck squamous cell carcinomas cell lines.BMC Cancer. 2016 Jul 8;16:431. doi: 10.1186/s12885-016-2468-x. BMC Cancer. 2016. PMID: 27391030 Free PMC article.
-
CoMEt: a statistical approach to identify combinations of mutually exclusive alterations in cancer.Genome Biol. 2015 Aug 8;16(1):160. doi: 10.1186/s13059-015-0700-7. Genome Biol. 2015. PMID: 26253137 Free PMC article.
-
Role of p38γ MAPK in regulation of EMT and cancer stem cells.Biochim Biophys Acta Mol Basis Dis. 2018 Nov;1864(11):3605-3617. doi: 10.1016/j.bbadis.2018.08.024. Epub 2018 Aug 18. Biochim Biophys Acta Mol Basis Dis. 2018. PMID: 30251680 Free PMC article.
References
-
- Hanahan D, Weinberg RA. Hallmarks of cancer: the next generation. Cell. 2011;144:646–674. - PubMed
-
- Hanahan D, Coussens LM. Accessories to the crime: functions of cells recruited to the tumor microenvironment. Cancer Cell. 2012;21:309–322. - PubMed
-
- Yang J, Weinberg RA. Epithelial-mesenchymal transition: at the crossroads of development and tumor metastasis. Dev. Cell. 2008;14:818–829. - PubMed
Publication types
MeSH terms
Substances
Grants and funding
LinkOut - more resources
Full Text Sources
Other Literature Sources
Medical
Molecular Biology Databases
Miscellaneous