Characterization of Fragile X Mental Retardation Protein granules formation and dynamics in Drosophila
- PMID: 23336078
- PMCID: PMC3545270
- DOI: 10.1242/bio.20123012
Characterization of Fragile X Mental Retardation Protein granules formation and dynamics in Drosophila
Abstract
FMRP is an evolutionarily conserved protein that is highly expressed in neurons and its deficiency causes fragile X mental retardation syndrome. FMRP controls the translation of target mRNAs in part by promoting their dynamic transport in neuronal RNA granules. We have previously shown that high expression of mammalian FMRP induces formation of granules termed FMRP granules. These RNA granules are reminiscent of neuronal granules, of stress granules, as well as of the recently described in vitro-assembled granules. In contrast with mammalian FMRP, which has two paralog proteins, Drosophila FMRP (dFMRP) is encoded by a single gene that has no paralog. Using this genetically simple organism, we investigated formation and dynamics of FMRP granules. We found that increased expression of dFMRP in Drosophila cells induces the formation of dynamic dFMRP RNA granules. Mutagenesis studies identified the N-terminal protein-protein domain of dFMRP as a key determinant for FMRP granules formation. The RGG RNA binding motif of dFMRP is dispensable for dFMRP granules formation since its deletion does not prevent formation of those granules. Deletion of the RGG motif reduced, however, dFMRP trafficking between FMRP granules and the cytosol. Similarly, deletion of a large part of the KH RNA binding motif of dFMRP had no effect on formation of dFMRP-granules, but diminished the shuttling activity of dFMRP. Our results thus suggest that the mechanisms controlling formation of RNA granules and those promoting their dynamics are uncoupled. This study opens new avenues to further elucidate the molecular mechanisms controlling FMRP trafficking with its associated mRNAs in and out of RNA granules.
Keywords: FMRP; Protein translation; RNA.
Figures
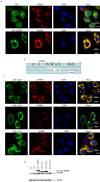
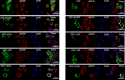
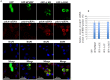
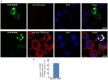
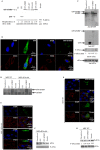
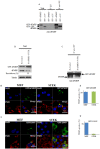
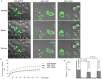
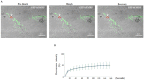
Similar articles
-
Characterization of fragile X mental retardation protein recruitment and dynamics in Drosophila stress granules.PLoS One. 2013;8(2):e55342. doi: 10.1371/journal.pone.0055342. Epub 2013 Feb 7. PLoS One. 2013. PMID: 23408971 Free PMC article.
-
Fragile X protein mitigates TDP-43 toxicity by remodeling RNA granules and restoring translation.Hum Mol Genet. 2015 Dec 15;24(24):6886-98. doi: 10.1093/hmg/ddv389. Epub 2015 Sep 18. Hum Mol Genet. 2015. PMID: 26385636 Free PMC article.
-
Proteomic analysis reveals CCT is a target of Fragile X mental retardation protein regulation in Drosophila.Dev Biol. 2010 Apr 15;340(2):408-18. doi: 10.1016/j.ydbio.2010.01.028. Epub 2010 Feb 1. Dev Biol. 2010. PMID: 20122915 Free PMC article.
-
Regulation of RNA granules by FMRP and implications for neurological diseases.Traffic. 2020 Jul;21(7):454-462. doi: 10.1111/tra.12733. Traffic. 2020. PMID: 32374065 Free PMC article. Review.
-
Cataloguing and Selection of mRNAs Localized to Dendrites in Neurons and Regulated by RNA-Binding Proteins in RNA Granules.Biomolecules. 2020 Jan 22;10(2):167. doi: 10.3390/biom10020167. Biomolecules. 2020. PMID: 31978946 Free PMC article. Review.
Cited by
-
Folding Mechanism and Aggregation Propensity of the KH0 Domain of FMRP and Its R138Q Pathological Variant.Int J Mol Sci. 2022 Oct 12;23(20):12178. doi: 10.3390/ijms232012178. Int J Mol Sci. 2022. PMID: 36293035 Free PMC article.
-
FMRP cooperates with miRISC components to repress translation and regulate neurite morphogenesis in Drosophila.RNA Biol. 2024 Jan;21(1):11-22. doi: 10.1080/15476286.2024.2392304. Epub 2024 Aug 27. RNA Biol. 2024. PMID: 39190491 Free PMC article.
-
Mechanistic insights into mammalian stress granule dynamics.J Cell Biol. 2016 Nov 7;215(3):313-323. doi: 10.1083/jcb.201609081. J Cell Biol. 2016. PMID: 27821493 Free PMC article. Review.
-
FMRP-PKA Activity Negative Feedback Regulates RNA Binding-Dependent Fibrillation in Brain Learning and Memory Circuitry.Cell Rep. 2020 Oct 13;33(2):108266. doi: 10.1016/j.celrep.2020.108266. Cell Rep. 2020. PMID: 33053340 Free PMC article.
-
Drosophila lines with mutant and wild type human TDP-43 replacing the endogenous gene reveals phosphorylation and ubiquitination in mutant lines in the absence of viability or lifespan defects.PLoS One. 2017 Jul 7;12(7):e0180828. doi: 10.1371/journal.pone.0180828. eCollection 2017. PLoS One. 2017. PMID: 28686708 Free PMC article.
References
-
- Barbee S. A., Estes P. S., Cziko A. M., Hillebrand J., Luedeman R. A., Coller J. M., Johnson N., Howlett I. C., Geng C., Ueda R.et al. (2006). Staufen- and FMRP-containing neuronal RNPs are structurally and functionally related to somatic P bodies. Neuron 52, 997–1009 10.1016/j.neuron.2006.10.028 - DOI - PMC - PubMed
LinkOut - more resources
Full Text Sources
Molecular Biology Databases
Miscellaneous