MSH6- or PMS2-deficiency causes re-replication in DT40 B cells, but it has little effect on immunoglobulin gene conversion or on repair of AID-generated uracils
- PMID: 23314153
- PMCID: PMC3597665
- DOI: 10.1093/nar/gks1470
MSH6- or PMS2-deficiency causes re-replication in DT40 B cells, but it has little effect on immunoglobulin gene conversion or on repair of AID-generated uracils
Abstract
The mammalian antibody repertoire is shaped by somatic hypermutation (SHM) and class switch recombination (CSR) of the immunoglobulin (Ig) loci of B lymphocytes. SHM and CSR are triggered by non-canonical, error-prone processing of G/U mismatches generated by activation-induced deaminase (AID). In birds, AID does not trigger SHM, but it triggers Ig gene conversion (GC), a 'homeologous' recombination process involving the Ig variable region and proximal pseudogenes. Because recombination fidelity is controlled by the mismatch repair (MMR) system, we investigated whether MMR affects GC in the chicken B cell line DT40. We show here that Msh6(-/-) and Pms2(-/-) DT40 cells display cell cycle defects, including genomic re-replication. However, although IgVλ GC tracts in MMR-deficient cells were slightly longer than in normal cells, Ig GC frequency, donor choice or the number of mutations per sequence remained unaltered. The finding that the avian MMR system, unlike that of mammals, does not seem to contribute towards the processing of G/U mismatches in vitro could explain why MMR is unable to initiate Ig GC in this species, despite initiating SHM and CSR in mammalian cells. Moreover, as MMR does not counteract or govern Ig GC, we report a rare example of 'homeologous' recombination insensitive to MMR.
Figures
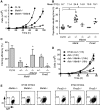
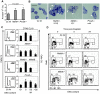
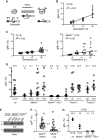
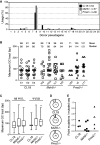
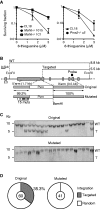
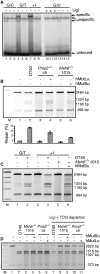
Similar articles
-
Chicken MBD4 Regulates Immunoglobulin Diversification by Somatic Hypermutation.Front Immunol. 2019 Nov 1;10:2540. doi: 10.3389/fimmu.2019.02540. eCollection 2019. Front Immunol. 2019. PMID: 31736964 Free PMC article.
-
Bcl6 Is Required for Somatic Hypermutation and Gene Conversion in Chicken DT40 Cells.PLoS One. 2016 Feb 22;11(2):e0149146. doi: 10.1371/journal.pone.0149146. eCollection 2016. PLoS One. 2016. PMID: 26900682 Free PMC article.
-
Somatic hypermutation and class switch recombination in Msh6(-/-)Ung(-/-) double-knockout mice.J Immunol. 2006 Oct 15;177(8):5386-92. doi: 10.4049/jimmunol.177.8.5386. J Immunol. 2006. PMID: 17015724
-
Mismatch-mediated error prone repair at the immunoglobulin genes.Biomed Pharmacother. 2011 Dec;65(8):529-36. doi: 10.1016/j.biopha.2011.09.001. Epub 2011 Oct 24. Biomed Pharmacother. 2011. PMID: 22100214 Free PMC article. Review.
-
Activation-induced cytidine deaminase-mediated hypermutation in the DT40 cell line.Philos Trans R Soc Lond B Biol Sci. 2009 Mar 12;364(1517):639-44. doi: 10.1098/rstb.2008.0202. Philos Trans R Soc Lond B Biol Sci. 2009. PMID: 19008193 Free PMC article. Review.
Cited by
-
DNA Damage Tolerance Mechanisms Revealed from the Analysis of Immunoglobulin V Gene Diversification in Avian DT40 Cells.Genes (Basel). 2018 Dec 7;9(12):614. doi: 10.3390/genes9120614. Genes (Basel). 2018. PMID: 30544644 Free PMC article. Review.
-
Positive Cofactor 4 (PC4) is critical for DNA repair pathway re-routing in DT40 cells.Sci Rep. 2016 Jul 4;6:28890. doi: 10.1038/srep28890. Sci Rep. 2016. PMID: 27374870 Free PMC article.
-
The uracil-DNA glycosylase UNG protects the fitness of normal and cancer B cells expressing AID.NAR Cancer. 2020 Aug 27;2(3):zcaa019. doi: 10.1093/narcan/zcaa019. eCollection 2020 Sep. NAR Cancer. 2020. PMID: 33554121 Free PMC article.
References
-
- Di Noia JM, Neuberger MS. Molecular mechanisms of antibody somatic hypermutation. Annu. Rev. Biochem. 2007;76:1–22. - PubMed
-
- Peled JU, Kuang FL, Iglesias-Ussel MD, Roa S, Kalis SL, Goodman MF, Scharff MD. The biochemistry of somatic hypermutation. Annu. Rev. Immunol. 2008;26:481–511. - PubMed
-
- Reynaud CA, Anquez V, Grimal H, Weill JC. A hyperconversion mechanism generates the chicken light chain preimmune repertoire. Cell. 1987;48:379–388. - PubMed
-
- Reynaud CA, Dahan A, Anquez V, Weill JC. Somatic hyperconversion diversifies the single Vh gene of the chicken with a high incidence in the D region. Cell. 1989;59:171–183. - PubMed
-
- Chaudhuri J, Alt FW. Class-switch recombination: interplay of transcription, DNA deamination and DNA repair. Nat. Rev. Immunol. 2004;4:541–552. - PubMed
Publication types
MeSH terms
Substances
Grants and funding
LinkOut - more resources
Full Text Sources
Other Literature Sources
Molecular Biology Databases
Miscellaneous