Eosinophil extracellular DNA trap cell death mediates lytic release of free secretion-competent eosinophil granules in humans
- PMID: 23303825
- PMCID: PMC3596967
- DOI: 10.1182/blood-2012-05-432088
Eosinophil extracellular DNA trap cell death mediates lytic release of free secretion-competent eosinophil granules in humans
Abstract
Eosinophils release their granule proteins extracellularly through exocytosis, piecemeal degranulation, or cytolytic degranulation. Findings in diverse human eosinophilic diseases of intact extracellular eosinophil granules, either free or clustered, indicate that eosinophil cytolysis occurs in vivo, but the mechanisms and consequences of lytic eosinophil degranulation are poorly understood. We demonstrate that activated human eosinophils can undergo extracellular DNA trap cell death (ETosis) that cytolytically releases free eosinophil granules. Eosinophil ETosis (EETosis), in response to immobilized immunoglobulins (IgG, IgA), cytokines with platelet activating factor, calcium ionophore, or phorbol myristate acetate, develops within 120 minutes in a reduced NADP (NADPH) oxidase-dependent manner. Initially, nuclear lobular formation is lost and some granules are released by budding off from the cell as plasma membrane-enveloped clusters. Following nuclear chromatolysis, plasma membrane lysis liberates DNA that forms weblike extracellular DNA nets and releases free intact granules. EETosis-released eosinophil granules, still retaining eosinophil cationic granule proteins, can be activated to secrete when stimulated with CC chemokine ligand 11 (eotaxin-1). Our results indicate that an active NADPH oxidase-dependent mechanism of cytolytic, nonapoptotic eosinophil death initiates nuclear chromatolysis that eventuates in the release of intact secretion-competent granules and the formation of extracellular DNA nets.
Figures
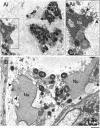
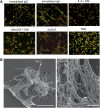
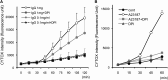
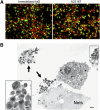
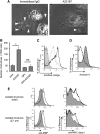
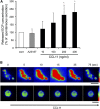
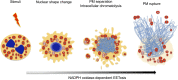
Similar articles
-
In Vivo ETosis of Human Eosinophils: The Ultrastructural Signature Captured by TEM in Eosinophilic Diseases.Front Immunol. 2022 Jul 7;13:938691. doi: 10.3389/fimmu.2022.938691. eCollection 2022. Front Immunol. 2022. PMID: 35874692 Free PMC article.
-
Vesicle-mediated secretion of human eosinophil granule-derived major basic protein.Lab Invest. 2009 Jul;89(7):769-81. doi: 10.1038/labinvest.2009.40. Epub 2009 Apr 27. Lab Invest. 2009. PMID: 19398958 Free PMC article.
-
CD63 is tightly associated with intracellular, secretory events chaperoning piecemeal degranulation and compound exocytosis in human eosinophils.J Leukoc Biol. 2016 Aug;100(2):391-401. doi: 10.1189/jlb.3A1015-480R. Epub 2016 Mar 10. J Leukoc Biol. 2016. PMID: 26965633 Free PMC article.
-
How to detect eosinophil ETosis (EETosis) and extracellular traps.Allergol Int. 2021 Jan;70(1):19-29. doi: 10.1016/j.alit.2020.10.002. Epub 2020 Nov 12. Allergol Int. 2021. PMID: 33189567 Free PMC article. Review.
-
Functional extracellular eosinophil granules: a bomb caught in a trap.Int Arch Allergy Immunol. 2013;162(4):276-82. doi: 10.1159/000354934. Epub 2013 Oct 17. Int Arch Allergy Immunol. 2013. PMID: 24136351 Review.
Cited by
-
Editorial: The role of leukocyte extracellular traps in Inflammation and autoimmunity.Front Immunol. 2022 Nov 1;13:1075026. doi: 10.3389/fimmu.2022.1075026. eCollection 2022. Front Immunol. 2022. PMID: 36389784 Free PMC article. No abstract available.
-
The Regulatory Function of Eosinophils.Microbiol Spectr. 2016 Oct;4(5):10.1128/microbiolspec.MCHD-0020-2015. doi: 10.1128/microbiolspec.MCHD-0020-2015. Microbiol Spectr. 2016. PMID: 27780017 Free PMC article. Review.
-
The Emerging Role of Eosinophils as Multifunctional Leukocytes in Health and Disease.Immune Netw. 2020 Jun 23;20(3):e24. doi: 10.4110/in.2020.20.e24. eCollection 2020 Jun. Immune Netw. 2020. PMID: 32655972 Free PMC article. Review.
-
Role of Mitochondria in the Regulation of Effector Functions of Granulocytes.Cells. 2023 Sep 5;12(18):2210. doi: 10.3390/cells12182210. Cells. 2023. PMID: 37759432 Free PMC article. Review.
-
Extracellular Trap by Blood Cells: Clinical Implications.Tissue Eng Regen Med. 2020 Apr;17(2):141-153. doi: 10.1007/s13770-020-00241-z. Epub 2020 Feb 29. Tissue Eng Regen Med. 2020. PMID: 32114678 Free PMC article. Review.
References
-
- Klion AD, Nutman TB. The role of eosinophils in host defense against helminth parasites. J Allergy Clin Immunol. 2004;113(1):30–37. - PubMed
-
- Kay AB. The role of eosinophils in the pathogenesis of asthma. Trends Mol Med. 2005;11(4):148–152. - PubMed
-
- Lee JJ, Lee NA. Eosinophil degranulation: an evolutionary vestige or a universally destructive effector function? Clin Exp Allergy. 2005;35(8):986–994. - PubMed
-
- Moqbel R, Coughlin JJ. Differential secretion of cytokines. Sci STKE. 2006;2006(338):pe26. - PubMed
Publication types
MeSH terms
Substances
Grants and funding
LinkOut - more resources
Full Text Sources
Other Literature Sources
Miscellaneous