Piperidinols that show anti-tubercular activity as inhibitors of arylamine N-acetyltransferase: an essential enzyme for mycobacterial survival inside macrophages
- PMID: 23285185
- PMCID: PMC3532304
- DOI: 10.1371/journal.pone.0052790
Piperidinols that show anti-tubercular activity as inhibitors of arylamine N-acetyltransferase: an essential enzyme for mycobacterial survival inside macrophages
Abstract
Latent M. tuberculosis infection presents one of the major obstacles in the global eradication of tuberculosis (TB). Cholesterol plays a critical role in the persistence of M. tuberculosis within the macrophage during latent infection. Catabolism of cholesterol contributes to the pool of propionyl-CoA, a precursor that is incorporated into cell-wall lipids. Arylamine N-acetyltransferase (NAT) is encoded within a gene cluster that is involved in the cholesterol sterol-ring degradation and is essential for intracellular survival. The ability of the NAT from M. tuberculosis (TBNAT) to utilise propionyl-CoA links it to the cholesterol-catabolism pathway. Deleting the nat gene or inhibiting the NAT enzyme prevents intracellular survival and results in depletion of cell-wall lipids. TBNAT has been investigated as a potential target for TB therapies. From a previous high-throughput screen, 3-benzoyl-4-phenyl-1-methylpiperidinol was identified as a selective inhibitor of prokaryotic NAT that exhibited antimycobacterial activity. The compound resulted in time-dependent irreversible inhibition of the NAT activity when tested against NAT from M. marinum (MMNAT). To further evaluate the antimycobacterial activity and the NAT inhibition of this compound, four piperidinol analogues were tested. All five compounds exert potent antimycobacterial activity against M. tuberculosis with MIC values of 2.3-16.9 µM. Treatment of the MMNAT enzyme with this set of inhibitors resulted in an irreversible time-dependent inhibition of NAT activity. Here we investigate the mechanism of NAT inhibition by studying protein-ligand interactions using mass spectrometry in combination with enzyme analysis and structure determination. We propose a covalent mechanism of NAT inhibition that involves the formation of a reactive intermediate and selective cysteine residue modification. These piperidinols present a unique class of antimycobacterial compounds that have a novel mode of action different from known anti-tubercular drugs.
Conflict of interest statement
Figures
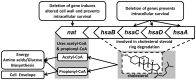
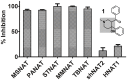
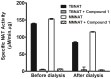
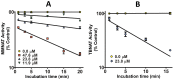
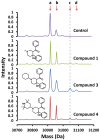
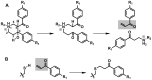
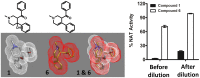
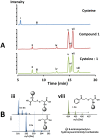
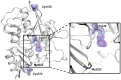
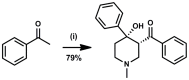
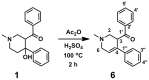
Similar articles
-
Probing the architecture of the Mycobacterium marinum arylamine N-acetyltransferase active site.Protein Cell. 2010 Apr;1(4):384-392. doi: 10.1007/s13238-010-0037-7. Epub 2010 May 8. Protein Cell. 2010. PMID: 21203950 Free PMC article.
-
Exploration of piperidinols as potential antitubercular agents.Molecules. 2014 Oct 10;19(10):16274-90. doi: 10.3390/molecules191016274. Molecules. 2014. PMID: 25310152 Free PMC article.
-
Identification of arylamine N-acetyltransferase inhibitors as an approach towards novel anti-tuberculars.Protein Cell. 2010 Jan;1(1):82-95. doi: 10.1007/s13238-010-0006-1. Epub 2010 Mar 18. Protein Cell. 2010. PMID: 21204000 Free PMC article.
-
Arylamine N-acetyltransferases in mycobacteria.Curr Drug Metab. 2008 Jul;9(6):510-9. doi: 10.2174/138920008784892100. Curr Drug Metab. 2008. PMID: 18680471 Free PMC article. Review.
-
Arylamine N-acetyltransferases: a pharmacogenomic approach to drug metabolism and endogenous function.Biochem Soc Trans. 2003 Jun;31(Pt 3):615-9. doi: 10.1042/bst0310615. Biochem Soc Trans. 2003. PMID: 12773167 Review.
Cited by
-
Arylamine N-acetyltransferases: from drug metabolism and pharmacogenetics to drug discovery.Br J Pharmacol. 2014 Jun;171(11):2705-25. doi: 10.1111/bph.12598. Br J Pharmacol. 2014. PMID: 24467436 Free PMC article. Review.
-
TB Summit 2014: prevention, diagnosis, and treatment of tuberculosis-a meeting report of a Euroscicon conference.Virulence. 2014 Jul 1;5(5):638-44. doi: 10.4161/viru.29803. Epub 2014 Jul 8. Virulence. 2014. PMID: 25003368 Free PMC article.
-
Xenobiotic-metabolizing enzymes in Bacillus anthracis: molecular and functional analysis of a truncated arylamine N-acetyltransferase isozyme.Br J Pharmacol. 2017 Jul;174(14):2174-2182. doi: 10.1111/bph.13647. Epub 2016 Nov 12. Br J Pharmacol. 2017. PMID: 27846346 Free PMC article.
-
New approaches to target the mycolic acid biosynthesis pathway for the development of tuberculosis therapeutics.Curr Pharm Des. 2014;20(27):4357-78. doi: 10.2174/1381612819666131118203641. Curr Pharm Des. 2014. PMID: 24245756 Free PMC article. Review.
-
Cryptic introgressions contribute to transgressive segregation for early blight resistance in tomato.Theor Appl Genet. 2021 Aug;134(8):2561-2575. doi: 10.1007/s00122-021-03842-x. Epub 2021 May 13. Theor Appl Genet. 2021. PMID: 33983452
References
-
- WHO (2011) World Health Organisation: Global tuberculosis control report 2011.
-
- Young DB, Gideon HP, Wilkinson RJ (2009) Eliminating latent tuberculosis. Trends in Microbiology 17: 183–188. - PubMed
Publication types
MeSH terms
Substances
Associated data
- Actions
Grants and funding
LinkOut - more resources
Full Text Sources