Transfer of extracellular vesicles during immune cell-cell interactions
- PMID: 23278745
- PMCID: PMC3740495
- DOI: 10.1111/imr.12013
Transfer of extracellular vesicles during immune cell-cell interactions
Abstract
The transfer of molecules between cells during cognate immune cell interactions has been reported, and recently a novel mechanism of transfer of proteins and genetic material such as small RNA between T cells and antigen-presenting cells (APCs) has been described, involving exchange of extracellular vesicles (EVs) during the formation of the immunological synapse (IS). EVs, a term that encompasses exosomes and microvesicles, has been implicated in cell-cell communication during immune responses associated with tumors, pathogens, allergies, and autoimmune diseases. This review focuses on EV transfer as a mechanism for the exchange of molecules during immune cell-cell interactions.
© 2012 John Wiley & Sons A/S. Published by Blackwell Publishing Ltd.
Figures
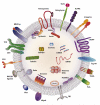
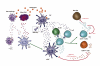
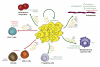
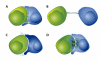
Similar articles
-
Unidirectional transfer of microRNA-loaded exosomes from T cells to antigen-presenting cells.Nat Commun. 2011;2:282. doi: 10.1038/ncomms1285. Nat Commun. 2011. PMID: 21505438 Free PMC article.
-
T Cell Microvilli: Sensors or Senders?Front Immunol. 2019 Jul 30;10:1753. doi: 10.3389/fimmu.2019.01753. eCollection 2019. Front Immunol. 2019. PMID: 31417549 Free PMC article. Review.
-
Dynamics of subsynaptic vesicles and surface microclusters at the immunological synapse.Sci Signal. 2010 May 11;3(121):ra36. doi: 10.1126/scisignal.2000645. Sci Signal. 2010. PMID: 20460647
-
Diversity in immunological synapse structure.Immunology. 2010 Dec;131(4):466-72. doi: 10.1111/j.1365-2567.2010.03366.x. Epub 2010 Oct 29. Immunology. 2010. PMID: 21039474 Free PMC article. Review.
-
Immunological synapse-driven transfer of extracellular vesicle microRNAs in primary lymphocytes.Methods Cell Biol. 2023;178:173-193. doi: 10.1016/bs.mcb.2022.12.004. Epub 2023 Jan 27. Methods Cell Biol. 2023. PMID: 37516525
Cited by
-
The effect of immune cell-derived exosomes in the cardiac tissue repair after myocardial infarction: Molecular mechanisms and pre-clinical evidence.J Cell Mol Med. 2021 Jul;25(14):6500-6510. doi: 10.1111/jcmm.16686. Epub 2021 Jun 5. J Cell Mol Med. 2021. PMID: 34092017 Free PMC article. Review.
-
The Role of Extracellular Vesicles in the Pathogenesis and Treatment of Autoimmune Disorders.Front Immunol. 2021 Feb 24;12:566299. doi: 10.3389/fimmu.2021.566299. eCollection 2021. Front Immunol. 2021. PMID: 33732229 Free PMC article. Review.
-
Genetic Background and Kinetics Define Wound Bed Extracellular Vesicles in a Mouse Model of Cutaneous Injury.Int J Mol Sci. 2021 Mar 29;22(7):3551. doi: 10.3390/ijms22073551. Int J Mol Sci. 2021. PMID: 33805585 Free PMC article.
-
Increased extracellular vesicles (EVs) related to T cell-mediated inflammation and vascular function in familial hypercholesterolemia.Atheroscler Plus. 2023 Jun 22;53:16-25. doi: 10.1016/j.athplu.2023.06.004. eCollection 2023 Sep. Atheroscler Plus. 2023. PMID: 37637934 Free PMC article.
-
Inhibitor of apoptosis proteins as therapeutic targets in multiple myeloma.Leukemia. 2014 Jul;28(7):1519-28. doi: 10.1038/leu.2014.2. Epub 2014 Jan 9. Leukemia. 2014. PMID: 24402161 Free PMC article.
References
-
- Thery C, Ostrowski M, Segura E. Membrane vesicles as conveyors of immune responses. Nat Rev Immunol. 2009;9:581–593. - PubMed
-
- Davis DM. Intercellular transfer of cell-surface proteins is common and can affect many stages of an immune response. Nat Rev Immunol. 2007;7:238–243. - PubMed
-
- Dustin ML. A novel adaptor protein orchestrates receptor patterning and cytoskeletal polarity in T-cell contacts. Cell. 1998;94:667–677. - PubMed
-
- Monks CR, Freiberg BA, Kupfer H, Sciaky N, Kupfer A. Three-dimensional segregation of supramolecular activation clusters in T cells. Nature. 1998;395:82–86. - PubMed
Publication types
MeSH terms
Substances
Grants and funding
LinkOut - more resources
Full Text Sources
Other Literature Sources
Miscellaneous