Host cell autophagy modulates early stages of adenovirus infections in airway epithelial cells
- PMID: 23236070
- PMCID: PMC3571477
- DOI: 10.1128/JVI.02014-12
Host cell autophagy modulates early stages of adenovirus infections in airway epithelial cells
Abstract
Human adenoviruses typically cause mild infections in the upper or lower respiratory tract, gastrointestinal tract, or ocular epithelium. However, adenoviruses may be life-threatening in patients with impaired immunity and some serotypes cause epidemic outbreaks. Attachment to host cell receptors activates cell signaling and virus uptake by endocytosis. At present, it is unclear how vital cellular homeostatic mechanisms affect these early steps in the adenovirus life cycle. Autophagy is a lysosomal degradation pathway for recycling intracellular components that is upregulated during periods of cell stress. Autophagic cargo is sequestered in double-membrane structures called autophagosomes that fuse with endosomes to form amphisomes which then deliver their content to lysosomes. Autophagy is an important adaptive response in airway epithelial cells targeted by many common adenovirus serotypes. Using two established tissue culture models, we demonstrate here that adaptive autophagy enhances expression of the early region 1 adenovirus protein, induction of mitogen-activated protein kinase signaling, and production of new viral progeny in airway epithelial cells infected with adenovirus type 2. We have also discovered that adenovirus infections are tightly regulated by endosome maturation, a process characterized by abrupt exchange of Rab5 and Rab7 GTPases, associated with early and late endosomes, respectively. Moreover, endosome maturation appears to control a pool of early endosomes capable of fusing with autophagosomes which enhance adenovirus infection. Many viruses have evolved mechanisms to induce autophagy in order to aid their own replication. Our studies reveal a novel role for host cell autophagy that could have a significant impact on the outcome of respiratory infections.
Figures
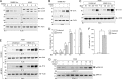
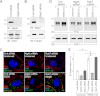
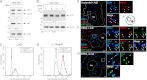
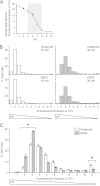
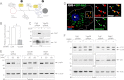
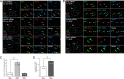
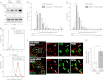
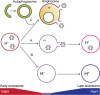
Similar articles
-
Adenovirus early region 3 RIDα protein limits NFκB signaling through stress-activated EGF receptors.PLoS Pathog. 2019 Aug 19;15(8):e1008017. doi: 10.1371/journal.ppat.1008017. eCollection 2019 Aug. PLoS Pathog. 2019. PMID: 31425554 Free PMC article.
-
Adenovirus Modulates Toll-Like Receptor 4 Signaling by Reprogramming ORP1L-VAP Protein Contacts for Cholesterol Transport from Endosomes to the Endoplasmic Reticulum.J Virol. 2017 Feb 28;91(6):e01904-16. doi: 10.1128/JVI.01904-16. Print 2017 Mar 15. J Virol. 2017. PMID: 28077646 Free PMC article.
-
Adenovirus Co-Opts Neutrophilic Inflammation to Enhance Transduction of Epithelial Cells.Viruses. 2021 Dec 22;14(1):13. doi: 10.3390/v14010013. Viruses. 2021. PMID: 35062217 Free PMC article.
-
Adenoviral inhibitors of apoptotic cell death.Virus Res. 2002 Sep;88(1-2):87-101. doi: 10.1016/s0168-1702(02)00122-3. Virus Res. 2002. PMID: 12297329 Review.
-
Understanding amphisomes.Biochem J. 2021 May 28;478(10):1959-1976. doi: 10.1042/BCJ20200917. Biochem J. 2021. PMID: 34047789 Free PMC article. Review.
Cited by
-
Innate immunity to adenovirus.Hum Gene Ther. 2014 Apr;25(4):265-84. doi: 10.1089/hum.2014.001. Epub 2014 Apr 8. Hum Gene Ther. 2014. PMID: 24512150 Free PMC article. Review.
-
Airway tight junctions as targets of viral infections.Tissue Barriers. 2021 Apr 3;9(2):1883965. doi: 10.1080/21688370.2021.1883965. Epub 2021 Feb 26. Tissue Barriers. 2021. PMID: 33632074 Free PMC article.
-
Adenovirus Reveals New Pathway for Cholesterol Egress from the Endolysosomal System.Int J Mol Sci. 2020 Aug 13;21(16):5808. doi: 10.3390/ijms21165808. Int J Mol Sci. 2020. PMID: 32823559 Free PMC article. Review.
-
Beclin 1 is required for neuron viability and regulates endosome pathways via the UVRAG-VPS34 complex.PLoS Genet. 2014 Oct 2;10(10):e1004626. doi: 10.1371/journal.pgen.1004626. eCollection 2014 Oct. PLoS Genet. 2014. PMID: 25275521 Free PMC article.
-
Multi-layered control of Galectin-8 mediated autophagy during adenovirus cell entry through a conserved PPxY motif in the viral capsid.PLoS Pathog. 2017 Feb 13;13(2):e1006217. doi: 10.1371/journal.ppat.1006217. eCollection 2017 Feb. PLoS Pathog. 2017. PMID: 28192531 Free PMC article.
References
-
- Horwitz M. 1995. Fields virology, 3rd ed Lippincott-Raven, Philadelphia, PA
-
- Paolino K, Sande J, Perez E, Loechelt B, Jantausch B, Painter W, Anderson M, Tippin T, Lanier ER, Fry T, DeBiasi RL. 2011. Eradication of disseminated adenovirus infection in a pediatric hematopoietic stem cell transplantation recipient using the novel antiviral agent CMX001. J. Clin. Virol. 50:167–170 - PubMed
-
- Bachtarzi H, Stevenson M, Fisher K. 2008. Cancer gene therapy with targeted adenoviruses. Expert Opin. Drug Deliv. 5:1231–1240 - PubMed
-
- Franz A, Adams O, Willems R, Bonzel L, Neuhausen N, Schweizer-Krantz S, Ruggeberg JU, Willers R, Henrich B, Schroten H, Tenenbaum T. 2010. Correlation of viral load of respiratory pathogens and co-infections with disease severity in children hospitalized for lower respiratory tract infection. J. Clin. Virol. 48:239–245 - PMC - PubMed
Publication types
MeSH terms
Grants and funding
LinkOut - more resources
Full Text Sources
Research Materials