Umbilical cord blood-derived mesenchymal stem cells inhibit, but adipose tissue-derived mesenchymal stem cells promote, glioblastoma multiforme proliferation
- PMID: 23231075
- PMCID: PMC3696928
- DOI: 10.1089/scd.2012.0486
Umbilical cord blood-derived mesenchymal stem cells inhibit, but adipose tissue-derived mesenchymal stem cells promote, glioblastoma multiforme proliferation
Abstract
Mesenchymal stem cells (MSCs) possess self-renewal and multipotential differentiation abilities, and they are thought to be one of the most reliable stem cell sources for a variety of cell therapies. Recently, cell therapy using MSCs has been studied as a novel therapeutic approach for cancers that show refractory progress and poor prognosis. MSCs from different tissues have different properties. However, the effect of different MSC properties on their application in anticancer therapies has not been thoroughly investigated. In this study, to characterize the anticancer therapeutic application of MSCs from different sources, we established two different kinds of human MSCs: umbilical cord blood-derived MSCs (UCB-MSCs) and adipose-tissue-derived MSCs (AT-MSCs). We used these MSCs in a coculture assay with primary glioblastoma multiforme (GBM) cells to analyze how MSCs from different sources can inhibit GBM growth. We found that UCB-MSCs inhibited GBM growth and caused apoptosis, but AT-MSCs promoted GBM growth. Terminal deoxynucleotidyl transferase-mediated biotinylated UTP nick-end labeling assay clearly demonstrated that UCB-MSCs promoted apoptosis of GBM via tumor necrosis factor-related apoptosis-inducing ligand (TRAIL). TRAIL was expressed more highly by UCB-MSCs than by AT-MSCs. Higher mRNA expression levels of angiogenic factors (vascular endothelial growth factor, angiopoietin 1, platelet-derived growth factor, and insulin-like growth factor) and stromal-derived factor-1 (SDF-1/CXCL12) were observed in AT-MSCs, and highly vascularized tumors were developed when AT-MSCs and GBM were cotransplanted. Importantly, CXCL12 inhibited TRAIL activation of the apoptotic pathway in GBM, suggesting that AT-MSCs may support GBM development in vivo by at least two distinct mechanisms-promoting angiogenesis and inhibiting apoptosis. The opposite effects of AT-MSCs and UCB-MSCs on GBM clearly demonstrate that differences must be considered when choosing a stem cell source for safety in clinical application.
Figures
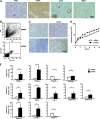
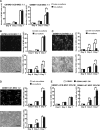
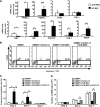
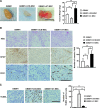
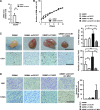
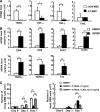
Similar articles
-
Gene therapy using TRAIL-secreting human umbilical cord blood-derived mesenchymal stem cells against intracranial glioma.Cancer Res. 2008 Dec 1;68(23):9614-23. doi: 10.1158/0008-5472.CAN-08-0451. Cancer Res. 2008. PMID: 19047138
-
Mesenchymal stem cells enhance the oncolytic effect of Newcastle disease virus in glioma cells and glioma stem cells via the secretion of TRAIL.Stem Cell Res Ther. 2016 Oct 10;7(1):149. doi: 10.1186/s13287-016-0414-0. Stem Cell Res Ther. 2016. PMID: 27724977 Free PMC article.
-
Tropism of mesenchymal stem cell toward CD133+ stem cell of glioblastoma in vitro and promote tumor proliferation in vivo.Stem Cell Res Ther. 2018 Nov 9;9(1):310. doi: 10.1186/s13287-018-1049-0. Stem Cell Res Ther. 2018. PMID: 30413179 Free PMC article.
-
Mesenchymal stem cells in glioblastoma therapy and progression: How one cell does it all.Biochim Biophys Acta Rev Cancer. 2021 Aug;1876(1):188582. doi: 10.1016/j.bbcan.2021.188582. Epub 2021 Jun 16. Biochim Biophys Acta Rev Cancer. 2021. PMID: 34144129 Review.
-
Cross talk between mesenchymal and glioblastoma stem cells: Communication beyond controversies.Stem Cells Transl Med. 2020 Nov;9(11):1310-1330. doi: 10.1002/sctm.20-0161. Epub 2020 Jun 15. Stem Cells Transl Med. 2020. PMID: 32543030 Free PMC article. Review.
Cited by
-
Recent Advances on Drug-Loaded Mesenchymal Stem Cells With Anti-neoplastic Agents for Targeted Treatment of Cancer.Front Bioeng Biotechnol. 2020 Jul 23;8:748. doi: 10.3389/fbioe.2020.00748. eCollection 2020. Front Bioeng Biotechnol. 2020. PMID: 32793565 Free PMC article. Review.
-
Interplay between mesenchymal stem cell and tumor and potential application.Hum Cell. 2020 Jul;33(3):444-458. doi: 10.1007/s13577-020-00369-z. Epub 2020 May 6. Hum Cell. 2020. PMID: 32378164 Review.
-
Xiaotan Sanjie decoction attenuates tumor angiogenesis by manipulating Notch-1-regulated proliferation of gastric cancer stem-like cells.World J Gastroenterol. 2014 Sep 28;20(36):13105-18. doi: 10.3748/wjg.v20.i36.13105. World J Gastroenterol. 2014. PMID: 25278704 Free PMC article.
-
MiR-134-5p/Stat3 Axis Modulates Proliferation and Migration of MSCs Co-Cultured with Glioma C6 Cells by Regulating Pvt1 Expression.Life (Basel). 2022 Oct 20;12(10):1648. doi: 10.3390/life12101648. Life (Basel). 2022. PMID: 36295083 Free PMC article.
-
Mesenchymal Stem Cell-Derived Extracellular Vesicles Increase Human MCF7 Breast Cancer Cell Proliferation associated with OCT4 Expression and ALDH Activity.Asian Pac J Cancer Prev. 2023 Aug 1;24(8):2781-2789. doi: 10.31557/APJCP.2023.24.8.2781. Asian Pac J Cancer Prev. 2023. PMID: 37642065 Free PMC article.
References
-
- Pittenger MF. Mackay AM. Beck SC. Jaiswal RK. Douglas R. Mosca JD. Moorman MA. Simonetti DW. Craig S. Marshak DR. Multilineage potential of adult human mesenchymal stem cells. Science. 1999;284:143–147. - PubMed
-
- Kern S. Eichler H. Stoeve J. Kluter H. Bieback K. Comparative analysis of mesenchymal stem cells from bone marrow, umbilical cord blood, or adipose tissue. Stem Cells. 2006;24:1294–1301. - PubMed
-
- Zuk PA. Zhu M. Mizuno H. Huang J. Futrell JW. Katz AJ. Benhaim P. Lorenz HP. Hedrick MH. Multilineage cells from human adipose tissue: implications for cell-based therapies. Tissue Eng. 2001;7:211–228. - PubMed
-
- Asakura A. Komaki M. Rudnicki M. Muscle satellite cells are multipotential stem cells that exhibit myogenic, osteogenic, and adipogenic differentiation. Differentiation. 2001;68:245–253. - PubMed
-
- De Bari C. Dell'Accio F. Tylzanowski P. Luyten FP. Multipotent mesenchymal stem cells from adult human synovial membrane. Arthritis Rheum. 2001;44:1928–1942. - PubMed
Publication types
MeSH terms
Substances
LinkOut - more resources
Full Text Sources
Other Literature Sources
Medical