Stable intronic sequence RNA (sisRNA), a new class of noncoding RNA from the oocyte nucleus of Xenopus tropicalis
- PMID: 23154985
- PMCID: PMC3505824
- DOI: 10.1101/gad.202184.112
Stable intronic sequence RNA (sisRNA), a new class of noncoding RNA from the oocyte nucleus of Xenopus tropicalis
Abstract
To compare nuclear and cytoplasmic RNA from a single cell type, free of cross-contamination, we studied the oocyte of the frog Xenopus tropicalis, a giant cell with an equally giant nucleus. We isolated RNA from manually dissected nuclei and cytoplasm of mature oocytes and subjected it to deep sequencing. Cytoplasmic mRNA consisted primarily of spliced exons derived from ∼6700 annotated genes. Nearly all of these genes were represented in the nucleus by intronic sequences. However, unspliced nascent transcripts were not detected. Inhibition of transcription or splicing for 1-2 d had little or no effect on the abundance of nuclear intronic sequences, demonstrating that they are unusually stable. RT-PCR analysis showed that these stable intronic sequences are transcribed from the coding strand and that a given intron can be processed into more than one molecule. Stable intronic sequence RNA (sisRNA) from the oocyte nucleus constitutes a new class of noncoding RNA. sisRNA is detectable by RT-PCR in samples of total RNA from embryos up to the mid-blastula stage, when zygotic transcription begins. Storage of sisRNA in the oocyte nucleus and its transmission to the developing embryo suggest that it may play important regulatory roles during oogenesis and/or early embryogenesis.
Figures
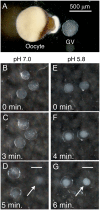
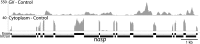
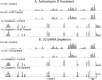
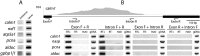
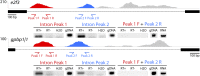
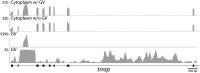
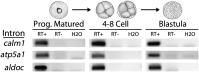
Similar articles
-
Lariat intronic RNAs in the cytoplasm of vertebrate cells.Proc Natl Acad Sci U S A. 2018 Aug 21;115(34):E7970-E7977. doi: 10.1073/pnas.1808816115. Epub 2018 Aug 6. Proc Natl Acad Sci U S A. 2018. PMID: 30082412 Free PMC article.
-
Lariat intronic RNAs in the cytoplasm of Xenopus tropicalis oocytes.RNA. 2014 Sep;20(9):1476-87. doi: 10.1261/rna.045781.114. Epub 2014 Jul 22. RNA. 2014. PMID: 25051970 Free PMC article.
-
Stable intronic sequence RNAs (sisRNAs) are selected regions in introns with distinct properties.BMC Genomics. 2020 Apr 7;21(1):287. doi: 10.1186/s12864-020-6687-9. BMC Genomics. 2020. PMID: 32264855 Free PMC article.
-
Circular sisRNA identification and characterisation.Methods. 2021 Dec;196:138-146. doi: 10.1016/j.ymeth.2021.04.002. Epub 2021 Apr 8. Methods. 2021. PMID: 33838268 Review.
-
Pre-mRNA splicing in the nuclei of Xenopus oocytes.Methods Mol Biol. 2006;322:149-63. doi: 10.1007/978-1-59745-000-3_11. Methods Mol Biol. 2006. PMID: 16739722 Review.
Cited by
-
A cluster of noncoding RNAs activates the ESR1 locus during breast cancer adaptation.Nat Commun. 2015 Apr 29;6:6966. doi: 10.1038/ncomms7966. Nat Commun. 2015. PMID: 25923108 Free PMC article.
-
Lariat intronic RNAs in the cytoplasm of vertebrate cells.Proc Natl Acad Sci U S A. 2018 Aug 21;115(34):E7970-E7977. doi: 10.1073/pnas.1808816115. Epub 2018 Aug 6. Proc Natl Acad Sci U S A. 2018. PMID: 30082412 Free PMC article.
-
Intron-lariat spliceosomes convert lariats to true circles: implications for intron transposition.bioRxiv [Preprint]. 2024 Mar 27:2024.03.26.586863. doi: 10.1101/2024.03.26.586863. bioRxiv. 2024. Update in: Genes Dev. 2024 May 21;38(7-8):322-335. doi: 10.1101/gad.351764.124 PMID: 38585890 Free PMC article. Updated. Preprint.
-
Intron lariat spliceosomes convert lariats to true circles: implications for intron transposition.Genes Dev. 2024 May 21;38(7-8):322-335. doi: 10.1101/gad.351764.124. Genes Dev. 2024. PMID: 38724209 Free PMC article.
-
Sex-specific trans-regulatory variation on the Drosophila melanogaster X chromosome.PLoS Genet. 2015 Feb 13;11(2):e1005015. doi: 10.1371/journal.pgen.1005015. eCollection 2015 Feb. PLoS Genet. 2015. PMID: 25679222 Free PMC article.
References
-
- Anderson DM, Smith LD 1978. Patterns of synthesis and accumulation of heterogeneous RNA in lampbrush stage oocytes of Xenopus laevis (Daudin). Dev Biol 67: 274–285 - PubMed
-
- Black DL 2003. Mechanisms of alternative pre-messenger RNA splicing. Annu Rev Biochem 72: 291–336 - PubMed
-
- Callan HG. 1986 Lampbrush chromosomes. Springer-Verlag, Berlin.
Publication types
MeSH terms
Substances
Grants and funding
LinkOut - more resources
Full Text Sources
Other Literature Sources
Molecular Biology Databases