Age-related changes in synaptic markers and monocyte subsets link the cognitive decline of APP(Swe)/PS1 mice
- PMID: 23125823
- PMCID: PMC3485573
- DOI: 10.3389/fncel.2012.00051
Age-related changes in synaptic markers and monocyte subsets link the cognitive decline of APP(Swe)/PS1 mice
Abstract
Alzheimer's disease (AD) is characterized by a progressive memory decline and numerous pathological abnormalities, including amyloid β (Aβ) accumulation in the brain and synaptic dysfunction. Here we wanted to study whether these brain changes were associated with alteration in the population of monocyte subsets since accumulating evidence supports the concept that the innate immune system plays a role in the etiology of this disease. We then determined the immune profile together with expression of genes encoding synaptic proteins and neurotrophins in APP(Swe)/PS1 mice and their age-matched wild-type (WT) littermates. We found that the progressive cognitive decline and the dramatic decrease in the expression of numerous synaptic markers and neurotrophins correlated with a major defect in the subset of circulating inflammatory monocytes. Indeed the number of CX(3)CR1(low)Ly6-C(high)CCR2(+)Gr1(+) monocytes remained essentially similar between 5 weeks and 6 months of age in APP(Swe)/PS1 mice, while these cells significantly increased in 6-month-old WT littermates. Of great interest is that the onset of cognitive decline was closely associated with the accumulation of soluble Aβ, disruption of synaptic activity, alteration in the BDNF system, and a defective production in the subset of CX(3)CR1(low)Ly6-C(high)CCR2(+)Gr1(+) monocytes. However, these memory impairments can be prevented or restored by boosting the monocytic production, using a short treatment of macrophage colony-stimulating factor (M-CSF). In conclusion, low CCR2(+) monocyte production by the hematopoietic system may be a direct biomarker of the cognitive decline in a context of AD.
Keywords: Alzheimer's disease; BDNF; CCR2; bone marrow-derived microglia; memory impairments; monocytes.
Figures
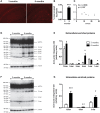
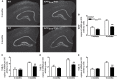
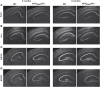
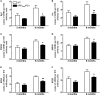
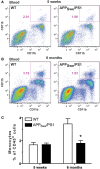
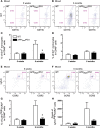
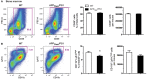
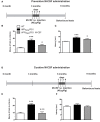
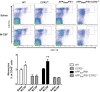
Similar articles
-
CC chemokine receptor 2 deficiency aggravates cognitive impairments and amyloid pathology in a transgenic mouse model of Alzheimer's disease.J Neurosci. 2011 Apr 20;31(16):6208-20. doi: 10.1523/JNEUROSCI.0299-11.2011. J Neurosci. 2011. PMID: 21508244 Free PMC article.
-
Hematopoietic CC-chemokine receptor 2 (CCR2) competent cells are protective for the cognitive impairments and amyloid pathology in a transgenic mouse model of Alzheimer's disease.Mol Med. 2012 Mar 30;18(1):297-313. doi: 10.2119/molmed.2011.00306. Mol Med. 2012. PMID: 22160221 Free PMC article.
-
Benzo(a)pyrene exposure induced neuronal loss, plaque deposition, and cognitive decline in APP/PS1 mice.J Neuroinflammation. 2020 Aug 31;17(1):258. doi: 10.1186/s12974-020-01925-y. J Neuroinflammation. 2020. PMID: 32867800 Free PMC article.
-
Powerful beneficial effects of macrophage colony-stimulating factor on beta-amyloid deposition and cognitive impairment in Alzheimer's disease.Brain. 2009 Apr;132(Pt 4):1078-92. doi: 10.1093/brain/awn331. Epub 2009 Jan 17. Brain. 2009. PMID: 19151372
-
Granulocyte colony stimulating factor decreases brain amyloid burden and reverses cognitive impairment in Alzheimer's mice.Neuroscience. 2009 Sep 29;163(1):55-72. doi: 10.1016/j.neuroscience.2009.05.071. Epub 2009 Jun 14. Neuroscience. 2009. PMID: 19500657 Free PMC article.
Cited by
-
Tissue-Plasminogen Activator Attenuates Alzheimer's Disease-Related Pathology Development in APPswe/PS1 Mice.Neuropsychopharmacology. 2016 Apr;41(5):1297-307. doi: 10.1038/npp.2015.279. Epub 2015 Sep 9. Neuropsychopharmacology. 2016. PMID: 26349911 Free PMC article.
-
Intravenous infusion of monocytes isolated from 2-week-old mice enhances clearance of Beta-amyloid plaques in an Alzheimer mouse model.PLoS One. 2015 Apr 1;10(4):e0121930. doi: 10.1371/journal.pone.0121930. eCollection 2015. PLoS One. 2015. PMID: 25830951 Free PMC article.
-
Multifocal Cerebral Microinfarcts Modulate Early Alzheimer's Disease Pathology in a Sex-Dependent Manner.Front Immunol. 2022 Jan 31;12:813536. doi: 10.3389/fimmu.2021.813536. eCollection 2021. Front Immunol. 2022. PMID: 35173711 Free PMC article.
-
The neuroimmune-neuroplasticity interface and brain pathology.Front Cell Neurosci. 2014 Dec 4;8:419. doi: 10.3389/fncel.2014.00419. eCollection 2014. Front Cell Neurosci. 2014. PMID: 25538568 Free PMC article. No abstract available.
-
Clearance of cerebral Aβ in Alzheimer's disease: reassessing the role of microglia and monocytes.Cell Mol Life Sci. 2017 Jun;74(12):2167-2201. doi: 10.1007/s00018-017-2463-7. Epub 2017 Feb 14. Cell Mol Life Sci. 2017. PMID: 28197669 Free PMC article. Review.
References
LinkOut - more resources
Full Text Sources
Molecular Biology Databases
Research Materials