N-WASP coordinates the delivery and F-actin-mediated capture of MT1-MMP at invasive pseudopods
- PMID: 23091069
- PMCID: PMC3483131
- DOI: 10.1083/jcb.201203025
N-WASP coordinates the delivery and F-actin-mediated capture of MT1-MMP at invasive pseudopods
Abstract
Metastasizing tumor cells use matrix metalloproteases, such as the transmembrane collagenase MT1-MMP, together with actin-based protrusions, to break through extracellular matrix barriers and migrate in dense matrix. Here we show that the actin nucleation-promoting protein N-WASP (Neural Wiskott-Aldrich syndrome protein) is up-regulated in breast cancer, and has a pivotal role in mediating the assembly of elongated pseudopodia that are instrumental in matrix degradation. Although a role for N-WASP in invadopodia was known, we now show how N-WASP regulates invasive protrusion in 3D matrices. In actively invading cells, N-WASP promoted trafficking of MT1-MMP into invasive pseudopodia, primarily from late endosomes, from which it was delivered to the plasma membrane. Upon MT1-MMP's arrival at the plasma membrane in pseudopodia, N-WASP stabilized MT1-MMP via direct tethering of its cytoplasmic tail to F-actin. Thus, N-WASP is crucial for extension of invasive pseudopods into which MT1-MMP traffics and for providing the correct cytoskeletal framework to couple matrix remodeling with protrusive invasion.
Figures
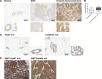
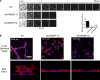
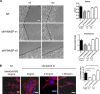
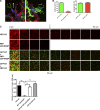
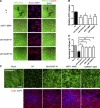
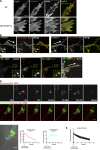
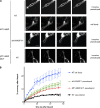
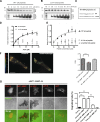
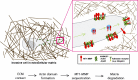
Similar articles
-
Control of MT1-MMP transport by atypical PKC during breast-cancer progression.Proc Natl Acad Sci U S A. 2014 May 6;111(18):E1872-9. doi: 10.1073/pnas.1400749111. Epub 2014 Apr 21. Proc Natl Acad Sci U S A. 2014. PMID: 24753582 Free PMC article.
-
CDCP1 regulates the function of MT1-MMP and invadopodia-mediated invasion of cancer cells.Mol Cancer Res. 2013 Jun;11(6):628-37. doi: 10.1158/1541-7786.MCR-12-0544. Epub 2013 Feb 25. Mol Cancer Res. 2013. PMID: 23439492
-
Cdc42-interacting protein 4 promotes breast cancer cell invasion and formation of invadopodia through activation of N-WASp.Cancer Res. 2010 Nov 1;70(21):8347-56. doi: 10.1158/0008-5472.CAN-09-4149. Epub 2010 Oct 12. Cancer Res. 2010. PMID: 20940394 Free PMC article.
-
Matrix invasion by tumour cells: a focus on MT1-MMP trafficking to invadopodia.J Cell Sci. 2009 Sep 1;122(Pt 17):3015-24. doi: 10.1242/jcs.034561. J Cell Sci. 2009. PMID: 19692588 Review.
-
Cellular and Molecular Mechanisms of MT1-MMP-Dependent Cancer Cell Invasion.Annu Rev Cell Dev Biol. 2016 Oct 6;32:555-576. doi: 10.1146/annurev-cellbio-111315-125227. Epub 2016 Aug 8. Annu Rev Cell Dev Biol. 2016. PMID: 27501444 Review.
Cited by
-
An epigenetically distinct breast cancer cell subpopulation promotes collective invasion.J Clin Invest. 2015 May;125(5):1927-43. doi: 10.1172/JCI77767. Epub 2015 Apr 6. J Clin Invest. 2015. PMID: 25844900 Free PMC article.
-
Sorting nexin 9 negatively regulates invadopodia formation and function in cancer cells.J Cell Sci. 2016 Jul 15;129(14):2804-16. doi: 10.1242/jcs.188045. Epub 2016 Jun 8. J Cell Sci. 2016. PMID: 27278018 Free PMC article.
-
SNX9 promotes metastasis by enhancing cancer cell invasion via differential regulation of RhoGTPases.Mol Biol Cell. 2016 Mar 9;27(9):1409-19. doi: 10.1091/mbc.E16-02-0101. Online ahead of print. Mol Biol Cell. 2016. PMID: 26960793 Free PMC article.
-
Mechanical Cues Affect Migration and Invasion of Cells From Three Different Directions.Front Cell Dev Biol. 2020 Sep 17;8:583226. doi: 10.3389/fcell.2020.583226. eCollection 2020. Front Cell Dev Biol. 2020. PMID: 33043017 Free PMC article. Review.
-
RAB2A controls MT1-MMP endocytic and E-cadherin polarized Golgi trafficking to promote invasive breast cancer programs.EMBO Rep. 2016 Jul;17(7):1061-80. doi: 10.15252/embr.201642032. Epub 2016 Jun 2. EMBO Rep. 2016. PMID: 27255086 Free PMC article.
References
-
- Artym V.V., Zhang Y., Seillier-Moiseiwitsch F., Yamada K.M., Mueller S.C. 2006. Dynamic interactions of cortactin and membrane type 1 matrix metalloproteinase at invadopodia: defining the stages of invadopodia formation and function. Cancer Res. 66:3034–3043 10.1158/0008-5472.CAN-05-2177 - DOI - PubMed
Publication types
MeSH terms
Substances
Grants and funding
LinkOut - more resources
Full Text Sources
Other Literature Sources
Medical