TspanC8 tetraspanins regulate ADAM10/Kuzbanian trafficking and promote Notch activation in flies and mammals
- PMID: 23091066
- PMCID: PMC3483123
- DOI: 10.1083/jcb.201201133
TspanC8 tetraspanins regulate ADAM10/Kuzbanian trafficking and promote Notch activation in flies and mammals
Erratum in
-
Correction: TspanC8 tetraspanins regulate ADAM10/Kuzbanian trafficking and promote Notch activation in flies and mammals.J Cell Biol. 2016 May 23;213(4):495-6. doi: 10.1083/jcb.20120113304262016c. Epub 2016 May 16. J Cell Biol. 2016. PMID: 27185832 Free PMC article. No abstract available.
Abstract
The metalloprotease ADAM10/Kuzbanian catalyzes the ligand-dependent ectodomain shedding of Notch receptors and activates Notch. Here, we show that the human tetraspanins of the evolutionary conserved TspanC8 subfamily (Tspan5, Tspan10, Tspan14, Tspan15, Tspan17, and Tspan33) directly interact with ADAM10, regulate its exit from the endoplasmic reticulum, and that four of them regulate ADAM10 surface expression levels. In an independent RNAi screen in Drosophila, two TspanC8 genes were identified as Notch regulators. Functional analysis of the three Drosophila TspanC8 genes (Tsp3A, Tsp86D, and Tsp26D) indicated that these genes act redundantly to promote Notch signaling. During oogenesis, TspanC8 genes were up-regulated in border cells and regulated Kuzbanian distribution, Notch activity, and cell migration. Furthermore, the human TspanC8 tetraspanins Tspan5 and Tspan14 positively regulated ligand-induced ADAM10-dependent Notch1 signaling. We conclude that TspanC8 tetraspanins have a conserved function in the regulation of ADAM10 trafficking and activity, thereby positively regulating Notch receptor activation.
Figures
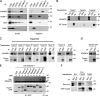
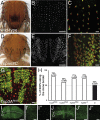
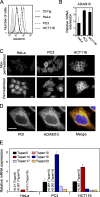
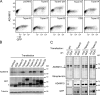
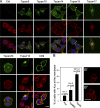
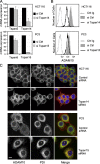
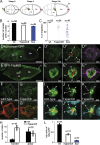
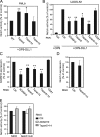
Similar articles
-
TspanC8 tetraspanins differentially regulate the cleavage of ADAM10 substrates, Notch activation and ADAM10 membrane compartmentalization.Cell Mol Life Sci. 2016 May;73(9):1895-915. doi: 10.1007/s00018-015-2111-z. Epub 2015 Dec 19. Cell Mol Life Sci. 2016. PMID: 26686862 Free PMC article.
-
TspanC8 Tetraspanins and A Disintegrin and Metalloprotease 10 (ADAM10) Interact via Their Extracellular Regions: EVIDENCE FOR DISTINCT BINDING MECHANISMS FOR DIFFERENT TspanC8 PROTEINS.J Biol Chem. 2016 Feb 12;291(7):3145-57. doi: 10.1074/jbc.M115.703058. Epub 2015 Dec 14. J Biol Chem. 2016. PMID: 26668317 Free PMC article.
-
TspanC8 tetraspanins differentially regulate ADAM10 endocytosis and half-life.Life Sci Alliance. 2019 Dec 2;3(1):e201900444. doi: 10.26508/lsa.201900444. Print 2020 Jan. Life Sci Alliance. 2019. PMID: 31792032 Free PMC article.
-
Regulation of the trafficking and the function of the metalloprotease ADAM10 by tetraspanins.Biochem Soc Trans. 2017 Aug 15;45(4):937-44. doi: 10.1042/BST20160296. Epub 2017 Jul 7. Biochem Soc Trans. 2017. PMID: 28687716 Review.
-
Regulation of ADAM10 by the TspanC8 Family of Tetraspanins and Their Therapeutic Potential.Int J Mol Sci. 2021 Jun 23;22(13):6707. doi: 10.3390/ijms22136707. Int J Mol Sci. 2021. PMID: 34201472 Free PMC article. Review.
Cited by
-
Decreased TSPAN14 Expression Contributes to NSCLC Progression.Life (Basel). 2022 Aug 23;12(9):1291. doi: 10.3390/life12091291. Life (Basel). 2022. PMID: 36143328 Free PMC article.
-
Tspan5 is an independent favourable prognostic factor and suppresses tumour growth in gastric cancer.Oncotarget. 2016 Jun 28;7(26):40160-40173. doi: 10.18632/oncotarget.9514. Oncotarget. 2016. PMID: 27223087 Free PMC article.
-
Tetraspanin 15 depletion impairs extracellular vesicle docking at target neurons.J Extracell Biol. 2023 Sep 11;2(9):e113. doi: 10.1002/jex2.113. eCollection 2023 Sep. J Extracell Biol. 2023. PMID: 38938373 Free PMC article.
-
Substrate-attached materials are enriched with tetraspanins and are analogous to the structures associated with rear-end retraction in migrating cells.Cell Adh Migr. 2013 May-Jun;7(3):304-14. doi: 10.4161/cam.25041. Epub 2013 May 15. Cell Adh Migr. 2013. PMID: 23676281 Free PMC article.
-
The cytoplasmic domain of a disintegrin and metalloproteinase 10 (ADAM10) regulates its constitutive activity but is dispensable for stimulated ADAM10-dependent shedding.J Biol Chem. 2015 Mar 20;290(12):7416-25. doi: 10.1074/jbc.M114.603753. Epub 2015 Jan 20. J Biol Chem. 2015. PMID: 25605720 Free PMC article.
References
-
- Arduise C., Abache T., Li L., Billard M., Chabanon A., Ludwig A., Mauduit P., Boucheix C., Rubinstein E., Le Naour F. 2008. Tetraspanins regulate ADAM10-mediated cleavage of TNF-alpha and epidermal growth factor. J. Immunol. 181:7002–7013. - PubMed
-
- Berditchevski F., Odintsova E., Sawada S., Gilbert E. 2002. Expression of the palmitoylation-deficient CD151 weakens the association of alpha 3 beta 1 integrin with the tetraspanin-enriched microdomains and affects integrin-dependent signaling. J. Biol. Chem. 277:36991–37000. 10.1074/jbc.M205265200 - DOI - PubMed
Publication types
MeSH terms
Substances
LinkOut - more resources
Full Text Sources
Molecular Biology Databases
Research Materials
Miscellaneous