The Par complex and integrins direct asymmetric cell division in adult intestinal stem cells
- PMID: 23040479
- PMCID: PMC3465556
- DOI: 10.1016/j.stem.2012.06.017
The Par complex and integrins direct asymmetric cell division in adult intestinal stem cells
Abstract
The adult Drosophila midgut is maintained by intestinal stem cells (ISCs) that generate both self-renewing and differentiating daughter cells. How this asymmetry is generated is currently unclear. Here, we demonstrate that asymmetric ISC division is established by a unique combination of extracellular and intracellular polarity mechanisms. We show that Integrin-dependent adhesion to the basement membrane induces cell-intrinsic polarity and results in the asymmetric segregation of the Par proteins Par-3, Par-6, and aPKC into the apical daughter cell. Cell-specific knockdown and overexpression experiments suggest that increased activity of aPKC enhances Delta/Notch signaling in one of the two daughter cells to induce terminal differentiation. Perturbing this mechanism or altering the orientation of ISC division results in the formation of intestinal tumors. Our data indicate that mechanisms for intrinsically asymmetric cell division can be adapted to allow for the flexibility in lineage decisions that is required in adult stem cells.
Copyright © 2012 Elsevier Inc. All rights reserved.
Figures
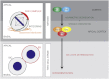
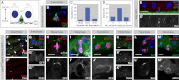
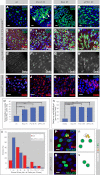
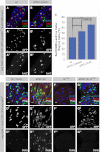
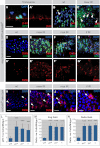
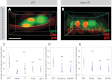
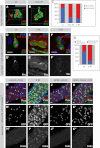
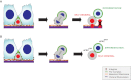
Similar articles
-
Sara endosomes and the asymmetric division of intestinal stem cells.Development. 2014 May;141(10):2014-23. doi: 10.1242/dev.104240. Development. 2014. PMID: 24803650
-
Cdc42 acts downstream of Bazooka to regulate neuroblast polarity through Par-6 aPKC.J Cell Sci. 2007 Sep 15;120(Pt 18):3200-6. doi: 10.1242/jcs.014902. Epub 2007 Aug 28. J Cell Sci. 2007. PMID: 17726059 Free PMC article.
-
Integrin signaling is required for maintenance and proliferation of intestinal stem cells in Drosophila.Dev Biol. 2013 May 1;377(1):177-87. doi: 10.1016/j.ydbio.2013.01.032. Epub 2013 Feb 8. Dev Biol. 2013. PMID: 23410794
-
The Par3/Par6/aPKC complex and epithelial cell polarity.Exp Cell Res. 2013 Jun 10;319(10):1357-64. doi: 10.1016/j.yexcr.2013.03.021. Epub 2013 Mar 25. Exp Cell Res. 2013. PMID: 23535009 Review.
-
Drosophila melanogaster Neuroblasts: A Model for Asymmetric Stem Cell Divisions.Results Probl Cell Differ. 2017;61:183-210. doi: 10.1007/978-3-319-53150-2_8. Results Probl Cell Differ. 2017. PMID: 28409305 Review.
Cited by
-
Intestinal stem cell response to injury: lessons from Drosophila.Cell Mol Life Sci. 2016 Sep;73(17):3337-49. doi: 10.1007/s00018-016-2235-9. Epub 2016 May 2. Cell Mol Life Sci. 2016. PMID: 27137186 Free PMC article. Review.
-
Control of Intestinal Cell Fate by Dynamic Mitotic Spindle Repositioning Influences Epithelial Homeostasis and Longevity.Cell Rep. 2019 Sep 10;28(11):2807-2823.e5. doi: 10.1016/j.celrep.2019.08.014. Cell Rep. 2019. PMID: 31509744 Free PMC article.
-
Identification of a new allele of O-fucosyltransferase 1 involved in Drosophila intestinal stem cell regulation.Biol Open. 2021 Nov 15;10(11):bio058910. doi: 10.1242/bio.058910. Epub 2021 Nov 3. Biol Open. 2021. PMID: 34731235 Free PMC article.
-
Opposing Post-transcriptional Control of InR by FMRP and LIN-28 Adjusts Stem Cell-Based Tissue Growth.Cell Rep. 2017 Dec 5;21(10):2671-2677. doi: 10.1016/j.celrep.2017.11.039. Cell Rep. 2017. PMID: 29212015 Free PMC article.
-
pRb-E2F signaling in life of mesenchymal stem cells: Cell cycle, cell fate, and cell differentiation.Genes Dis. 2014 Sep 30;1(2):174-187. doi: 10.1016/j.gendis.2014.09.007. eCollection 2014 Dec. Genes Dis. 2014. PMID: 30258863 Free PMC article. Review.
References
-
- Baumann O. Posterior midgut epithelial cells differ in their organization of the membrane skeleton from other drosophila epithelia. Exp. Cell Res. 2001;270:176–187. - PubMed
-
- Brower D.L., Jaffe S.M. Requirement for integrins during Drosophila wing development. Nature. 1989;342:285–287. - PubMed
-
- Brown N.H. Cell-cell adhesion via the ECM: integrin genetics in fly and worm. Matrix Biol. 2000;19:191–201. - PubMed
Publication types
MeSH terms
Substances
Grants and funding
LinkOut - more resources
Full Text Sources
Other Literature Sources
Molecular Biology Databases
Miscellaneous