Mutations in ADAR1 cause Aicardi-Goutières syndrome associated with a type I interferon signature
- PMID: 23001123
- PMCID: PMC4154508
- DOI: 10.1038/ng.2414
Mutations in ADAR1 cause Aicardi-Goutières syndrome associated with a type I interferon signature
Abstract
Adenosine deaminases acting on RNA (ADARs) catalyze the hydrolytic deamination of adenosine to inosine in double-stranded RNA (dsRNA) and thereby potentially alter the information content and structure of cellular RNAs. Notably, although the overwhelming majority of such editing events occur in transcripts derived from Alu repeat elements, the biological function of non-coding RNA editing remains uncertain. Here, we show that mutations in ADAR1 (also known as ADAR) cause the autoimmune disorder Aicardi-Goutières syndrome (AGS). As in Adar1-null mice, the human disease state is associated with upregulation of interferon-stimulated genes, indicating a possible role for ADAR1 as a suppressor of type I interferon signaling. Considering recent insights derived from the study of other AGS-related proteins, we speculate that ADAR1 may limit the cytoplasmic accumulation of the dsRNA generated from genomic repetitive elements.
Figures
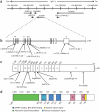
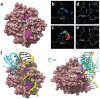
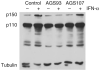
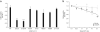
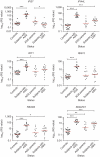
Similar articles
-
Aicardi-Goutières syndrome-associated mutation at ADAR1 gene locus activates innate immune response in mouse brain.J Neuroinflammation. 2021 Jul 31;18(1):169. doi: 10.1186/s12974-021-02217-9. J Neuroinflammation. 2021. PMID: 34332594 Free PMC article.
-
Human ADAR1 Prevents Endogenous RNA from Triggering Translational Shutdown.Cell. 2018 Feb 8;172(4):811-824.e14. doi: 10.1016/j.cell.2017.12.038. Epub 2018 Jan 25. Cell. 2018. PMID: 29395325 Free PMC article.
-
Mutations in the adenosine deaminase ADAR1 that prevent endogenous Z-RNA binding induce Aicardi-Goutières-syndrome-like encephalopathy.Immunity. 2021 Sep 14;54(9):1976-1988.e7. doi: 10.1016/j.immuni.2021.08.022. Immunity. 2021. PMID: 34525338
-
The role of RNA editing enzyme ADAR1 in human disease.Wiley Interdiscip Rev RNA. 2022 Jan;13(1):e1665. doi: 10.1002/wrna.1665. Epub 2021 Jun 8. Wiley Interdiscip Rev RNA. 2022. PMID: 34105255 Free PMC article. Review.
-
Effects of Aicardi-Goutières syndrome mutations predicted from ADAR-RNA structures.RNA Biol. 2017 Feb;14(2):164-170. doi: 10.1080/15476286.2016.1267097. Epub 2016 Dec 12. RNA Biol. 2017. PMID: 27937139 Free PMC article. Review.
Cited by
-
An overview of genetic mutations in Aicardi-Goutières syndrome in Iranian population.Neurol Sci. 2024 Oct 29. doi: 10.1007/s10072-024-07824-x. Online ahead of print. Neurol Sci. 2024. PMID: 39470906
-
A Fluorescent Adenosine Analogue as a Substrate for an A-to-I RNA Editing Enzyme.Angew Chem Int Ed Engl. 2015 Jul 20;54(30):8713-6. doi: 10.1002/anie.201502070. Epub 2015 Jun 10. Angew Chem Int Ed Engl. 2015. PMID: 26095193 Free PMC article.
-
The interactomes of influenza virus NS1 and NS2 proteins identify new host factors and provide insights for ADAR1 playing a supportive role in virus replication.PLoS Pathog. 2013;9(7):e1003440. doi: 10.1371/journal.ppat.1003440. Epub 2013 Jul 4. PLoS Pathog. 2013. PMID: 23853584 Free PMC article.
-
RNA Recognition and Immunity-Innate Immune Sensing and Its Posttranscriptional Regulation Mechanisms.Cells. 2020 Jul 16;9(7):1701. doi: 10.3390/cells9071701. Cells. 2020. PMID: 32708595 Free PMC article. Review.
-
[Genetics of lupus erythematosus].Hautarzt. 2015 Feb;66(2):121-8; quiz 129-30. doi: 10.1007/s00105-014-3570-0. Hautarzt. 2015. PMID: 25659384 German.
References
-
- Crow YJ, Livingston JH. Aicardi-Goutières syndrome: an important Mendelian mimic of congenital infection. Dev. Med. Child Neurol. 2008;50:410–416. - PubMed
-
- Lebon P, et al. Intrathecal synthesis of interferon-α in infants with progressive familial encephalopathy. J. Neurol. Sci. 1988;84:201–208. - PubMed
-
- Crow YJ, et al. Mutations in the gene encoding the 3′-5′ DNA exonuclease TREX1 cause Aicardi-Goutières syndrome at the AGS1 locus. Nat. Genet. 2006;38:917–920. - PubMed
-
- Crow YJ, et al. Mutations in genes encoding ribonuclease H2 subunits cause Aicardi-Goutières syndrome and mimic congenital viral brain infection. Nat. Genet. 2006;38:910–916. - PubMed
Publication types
MeSH terms
Substances
Supplementary concepts
Grants and funding
- RC2 HL102926/HL/NHLBI NIH HHS/United States
- RC2 HL102924/HL/NHLBI NIH HHS/United States
- HL-102926/HL/NHLBI NIH HHS/United States
- HL-102925/HL/NHLBI NIH HHS/United States
- RC2 HL103010/HL/NHLBI NIH HHS/United States
- HL-102923/HL/NHLBI NIH HHS/United States
- RC2 HL102923/HL/NHLBI NIH HHS/United States
- UC2 HL102926/HL/NHLBI NIH HHS/United States
- UC2 HL103010/HL/NHLBI NIH HHS/United States
- HL-103010/HL/NHLBI NIH HHS/United States
- K08 NS060695/NS/NINDS NIH HHS/United States
- HL-102924/HL/NHLBI NIH HHS/United States
- UC2 HL102923/HL/NHLBI NIH HHS/United States
- UC2 HL102924/HL/NHLBI NIH HHS/United States
- RC2 HL102925/HL/NHLBI NIH HHS/United States
- UC2 HL102925/HL/NHLBI NIH HHS/United States
LinkOut - more resources
Full Text Sources
Other Literature Sources
Medical
Molecular Biology Databases
Research Materials